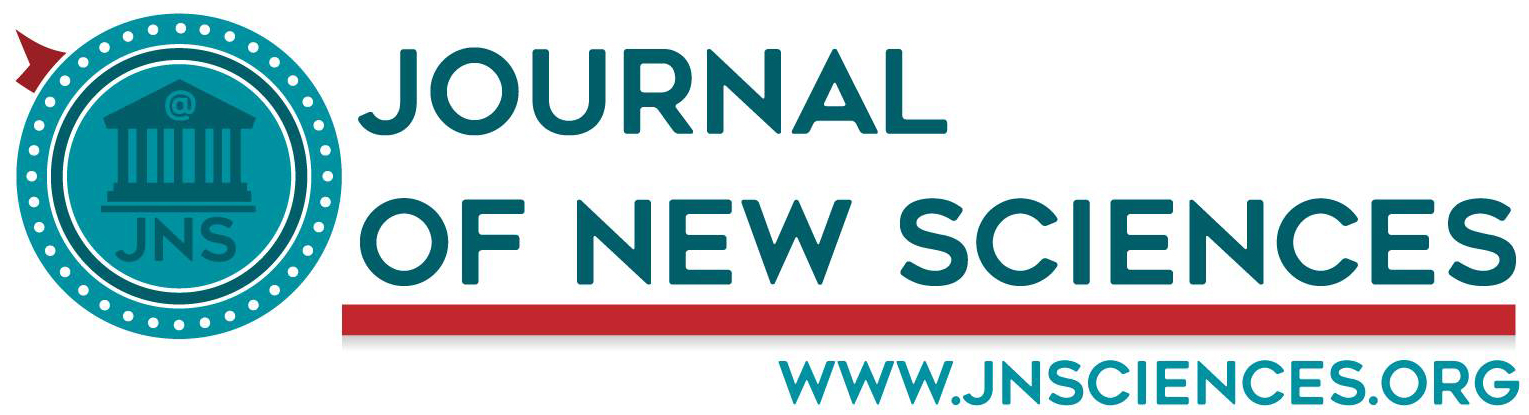
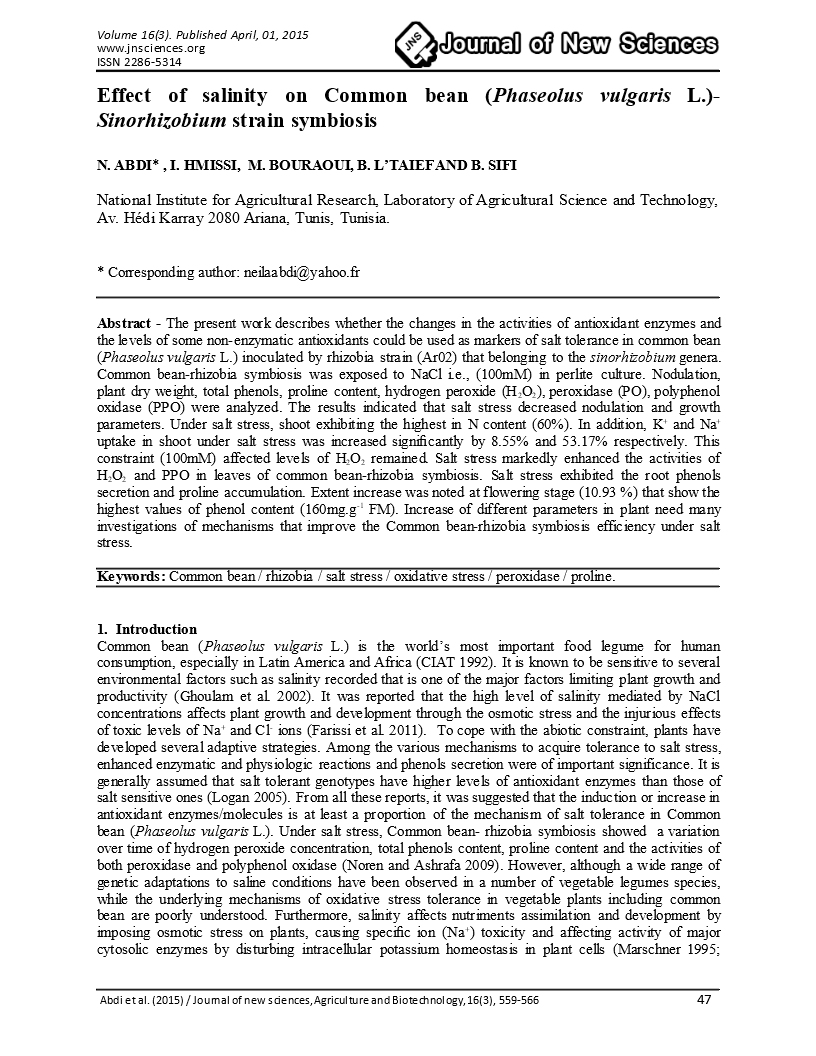
- Category: Volume 16
- Hits: 13572
Effect of salinity on Common bean (Phaseolus vulgaris L.)- Sinorhizobium strain symbiosis
N. Abdi*
I. Hmissi
M. Bouraoui
B. L’taief
B. Sifi
National Institute for Agricultural Research, Laboratory of Agricultural Science and Technology, Av. Hédi Karray 2080 Ariana, Tunis, Tunisia.
Abstract - The present work describes whether the changes in the activities of antioxidant enzymes and the levels of some non-enzymatic antioxidants could be used as markers of salt tolerance in common bean (Phaseolus vulgaris L.) inoculated by rhizobia strain (Ar02) that belonging to the sinorhizobium genera. Common bean-rhizobia symbiosis was exposed to NaCl i.e., (100mM) in perlite culture. Nodulation, plant dry weight, total phenols, proline content, hydrogen peroxide (H2O2), peroxidase (PO), polyphenol oxidase (PPO) were analyzed. The results indicated that salt stress decreased nodulation and growth parameters. Under salt stress, shoot exhibiting the highest in N content (60%). In addition, K+ and Na+ uptake in shoot under salt stress was increased significantly by 8.55% and 53.17% respectively. This constraint (100mM) affected levels of H2O2 remained. Salt stress markedly enhanced the activities of H2O2 and PPO in leaves of common bean-rhizobia symbiosis. Salt stress exhibited the root phenols secretion and proline accumulation. Extent increase was noted at flowering stage (10.93 %) that show the highest values of phenol content (160mg.g-1 FM). Increase of different parameters in plant need many investigations of mechanisms that improve the Common bean-rhizobia symbiosis efficiency under salt stress.
Keywords: Common bean / rhizobia / salt stress / oxidative stress / peroxidase / proline.
-
Introduction
Common bean (Phaseolus vulgaris L.) is the world’s most important food legume for human consumption, especially in Latin America and Africa (CIAT 1992). It is known to be sensitive to several environmental factors such as salinity recorded that is one of the major factors limiting plant growth and productivity (Ghoulam et al. 2002). It was reported that the high level of salinity mediated by NaCl concentrations affects plant growth and development through the osmotic stress and the injurious effects of toxic levels of Na+ and Cl- ions (Farissi et al. 2011). To cope with the abiotic constraint, plants have developed several adaptive strategies. Among the various mechanisms to acquire tolerance to salt stress, enhanced enzymatic and physiologic reactions and phenols secretion were of important significance. It is generally assumed that salt tolerant genotypes have higher levels of antioxidant enzymes than those of salt sensitive ones (Logan 2005). From all these reports, it was suggested that the induction or increase in antioxidant enzymes/molecules is at least a proportion of the mechanism of salt tolerance in Common bean (Phaseolus vulgaris L.). Under salt stress, Common bean- rhizobia symbiosis showed a variation over time of hydrogen peroxide concentration, total phenols content, proline content and the activities of both peroxidase and polyphenol oxidase (Noren and Ashrafa 2009). However, although a wide range of genetic adaptations to saline conditions have been observed in a number of vegetable legumes species, while the underlying mechanisms of oxidative stress tolerance in vegetable plants including common bean are poorly understood. Furthermore, salinity affects nutriments assimilation and development by imposing osmotic stress on plants, causing specific ion (Na+) toxicity and affecting activity of major cytosolic enzymes by disturbing intracellular potassium homeostasis in plant cells (Marschner 1995; Sairam and Srivastava 2002; Cuin and Shabala 2007; Chen et al. 2007). So that, the above effects take place in both roots and shoots tissues but operate in different timescales. Specific Na+ toxicity in leaves becomes critical only after many days (even weeks) after onset of salinity treatment (Munns and Tester 2008). Also, both massive depletion of cytosolic K+ in plant shoot and roots (Shabala et al. 2006). Additional, Cassman (1981) recommended that an adequate distribution of P between the shoots and roots was reported as an interesting parameter that may influence P use efficiency in N2 fixing symbiosis. Plants are known to involve several mechanisms to increase their P absorption efficiency (Kaous et al. 2009). Consequently, this study is among the first reports investigating morphological, physiological and oxidative metabolism changes in plant of N2-fixing Common bean under salt stress. Therefore, the major objectives of the present study were to assess the extent of regulation of various antioxidants and metabolites and whether these antioxidant metabolites show a positive association with the degree of salt pea tolerance.
-
Materials and methods
The seeds of Phaseolus vulgaris L. widespread agricultural use in Tunisia were inoculated separately with salt-tolerant strain nodulating Common bean in Tunisian soils belonging to the sinorhizobium genera. Seedlings were grown in controlled environmental, under greenhouse conditions with plant growth in pots. They were sterilised in 2% calcium hypochlorite, washed with sterile distilled water and germinated at 28 °C in soft agar containing 100 ml of Bergersen solution (Vincent 1970). The rhizobial inoculant was prepared from salt-tolerant rhizobia strains, preserved in tubes at 4 °C on YEM media (Vincent 1970). Rhizobia were grown in liquid YEM solution into an Erlenmeyer with agitation during 2 days at 28 °C, in darkness. Inoculation was performed by soaking 4-day-old seedlings with 100 ml of inoculants containing approximately 108 cells ml-1. The seedlings were transplanted into plastic pots containing 0.5 kg of the sterilised perlite. Afterwards, they were separated into two groups (four plants per treatment) and irrigated with nutrient solution supplemented with 0 (control), 100mM of NaCl. Plants were harvested at flowering stage of culture. They were separated into shoot, nodule and root components. They were dried at 70 ºC for 3 days to constant weight. Dry matter were then determined.
-
Determination of N and P contents in shoots and roots
Shoots and roots P were determined by the molybdate blue method (Murphy and Riley 1962). 0.3 g of dry weight were incinerated and filtrated (through Whatman No.1 filter paper) and a final volume taken up to 50 ml with distilled water. 100 μl of each extract was added to 2.5 ml of a reactive mixture prepared with ammonium molybdate (2.5%) and hydrazine sulfate (0.15%). Thereafter, absorbance was read at 820 nm after incubation at room temperature for 30 min. For N determination, 0.5 g shoot and root subsamples were used and analyzed by the Kjeldahl method.
-
Determination of K+ and Na+ contents in shoots and roots
The dry mass (0.3g) were incinerated and filtrated (through Whatman No.1 filter paper) and final volume was made up to 50 ml with distilled water. The determination of levels of Na+ and K+ was performed by a spectrophotometer Jenway flame types. The results were expressed as mg. Kg-1 dry weight.
-
Content of leaves and nodules H2O2
Leaves H2O2 content was determined according to the method of Velikova et al. (2000). An aliquot of 100 mg of leaf sample was ground in 2 ml of TCA (20 %) and then centrifuged at 15000g for 15 min at 4 ° C. The supernatants were then collected to determine the H2O2 content of these extracts. To 0.5 ml of the extract was added 0.5 ml of potassium phosphate buffer (10 mM, pH 7) and 1 ml of potassium iodine (1M) was then determined at 390 nm after 1 hour of incubation in the dark. The H2O2 content was expressed in micromol per g of fresh weight in reference to a standard prepared under the same conditions with known concentrations of H2O2 range.
-
Enzyme extractions and assays
The enzyme extract of peroxidase (PO) and polyphenol oxidase (PPO) was prepared at 0-4°C by homogenizing 100 mg of leaves samples with 10 % (w/w) polyvinyl polypyrrolidone and 1 mL of 0.1 M phosphate buffer (pH 7.0). The homogenate was centrifuged at 13000 rpm for 20 min at 4 °C and the supernatant was used to determine the activities of these enzymes (Tejera et al. 2004). The PO activity was determined according to Diani et al. (2009). The reaction mixture consisted of 200 µL of H2O2 at 0.3 %, 300 µL of guaiacol at 20 mM, 2 mL of phosphate buffer (0.1 M, pH 6), 1 mL of distilled water and 50 µL of enzymatic extract. PO activity was determined by following the decomposition of H2O2 at 470 nm. For PPO activity assay (Hori et al. 1997), the reaction mixture consisted of 500 µL catechol at 1.6 % in phosphate buffer (0.1 M, pH 6), 250 µL of distilled water, 200 µL of phosphate buffer (0.1 M, pH 6) and 100 µL of enzymatic extract. Thereafter the absorbance was recorded at 410 nm. For both enzymes, reading of the optical density was checked once every 60s during 3 min of incubation against a control where enzymatic extract was replaced by distilled water. Enzyme activities were expressed as the amount of protein decomposing 1 µmol of H2O2 per g FM.
-
Proline contents
The proline content was determined using the method described by Bates et al. (1973). Proline was extracted from leaves, roots and nodules samples of 100 mg fresh weight with 2 ml of 40% (methanol: water). The tubes were incubated in a water bath at 85 °C for 30 min, then 1 ml of extract was mixed with 1 ml of a mixture of glacial acetic acid and orthophosphoric acid (6 M) (3: 2; v/v) and 25 mg ninhydrin. After 1 h incubation at 100°C, the tubes were cooled and 5 ml of toluene was then added. The absorbance of the upper phase was spectrophotometrically determined at 528 nm. The proline concentration was determined using a standard curve obtained using reference proline solutions.
-
Total phenols content
Frozen roots from the different treatments were reduced to a fine powder using a pestle and a precooled mortar and were extracted three times with 80% methanol at 4°C under continuous stirring. The homogenate was centrifuged for 3 min and the supernatants were analyzed by spectrophotometer. Total phenols content was estimated based on the Foline-Ciocalteu method adapted from Dicko et al. (2002) and absorption at 760 nm was read using Cary100-UV spectrophotometer. A calibration curve was generated with freshly prepared solutions of (+)-catechin. Results were calculated as mg equivalent (+)-catechin per g of fresh weight (mg eq. (+)-catechin/g fresh weight (FM)).
-
Statistical analyses
The experiments were performed in a completely randomized design. All data were subjected to three-way analysis of variance (ANOVA) and the means were compared by LSD test at 1 % probability. Results are means of five replicates for both growth and nodulation and four replicates for all the remaining tested parameters. Data followed by the same letter are not significantly different at the level of 1 % probability.
-
Results and discussion
-
Plant growth and nodulation
-
Under salt stress, plant growth and nodulation were examined in Phaseolus vulgaris-rhizobia symbiosis. Obtained results revealed a significant decrease in biomass production of all examined symbiosis combination (Table 1). Similarly, Bargaz et al. (2013) and (Krouma et al. 2008) observed the same results under phosphorus and iron deficiency. Overall, salt decrease root length and root dry weigh; the decrease was in order to 5.88 %. The same idea was detected on nodule number and dry weights that were decreased in response to salt stress in P.vulgaris-rhizobia symbiosis (Table 1). Comparable type of observation was reported by Abdi et al. (2012).
Table 1. Phosphorus, potassium and sodium content in shoot and root of Common bean-rhizobia symbiosis grown under salt stress (100mM). Data are means and SE of four replicates harvested at flowering stage. |
||||||
Treatments |
Nodulation |
NDW (g/Pl.) |
Shoot lenght |
SDW (g/Pl.) |
Root Lenght |
RDW (g/Pl.) |
Control |
0±0.00 |
0±0.00 |
26.256±0.267 |
0.221±0.111 |
18.16±2.40 |
0.243±0.033 |
Ar02 |
1.6±2.07 |
0.02±0.02 |
26.754±0.613 |
0.366±0.190 |
20.4±2.12 |
0.266±0.036 |
Ar02+100mM |
0±0.00 |
0 |
26.130±0.210 |
0.318±0.120 |
19.2±3.11 |
0.28±0.035 |
Mean values labelled with the same letter were not significantly different at P<0.01. |
-
Shoot and Root N content
Interestingly, shoot N content increased significantly for all tested treatment (Figure 1). Shoot N content increase in order to 40%. The same results were observed with root N content. The decrease was in order to 75% under salt stress (Figure1). Although, 100mM NaCl supply accumulated significantly N in shoot and root of Phaseolus vulgaris L. inoculated with Ar02. Cordovilla et al. (1996) mentioned that plants dependent on nitrogen fixation are not always more sensitive to salinity. Under salt stress (100mM), shoot N content was three times more than in control treatment (Figure 1). Examination of oxidative stress in shoot and root is not only to advance understanding of salt stress tolerance in N2-fixing legumes, but also to point out this stress that has so far not been studied in common bean growth parameters up to now.
|
Figure 1: Nitrogen content in shoot and root of Common bean-rhizobia combinations grown under salt stress. Data are means and SE of five replicates harvested at flowering stage. |
-
Shoot and Root P, K+ and Na+ contents
Results showed in Table 2 that salt stress caused significant increase of phosphorus content in shoot and root of P.vulgaris L. - rhizobia symbiosis that was in order to 31.81% and 1.62% respectively. The same idea was observed with potassium and sodium content. Results showed significant increases of two elements on comparison to control treatment. Overall, salt stress severely increased P uptake and K+ and Na+ absorption (Table 2). In our study we did report a significative decrease in biomass after main salt treatment, shoot and root biomass was almost superior to control treatment and this was despite plants had higher K+ and Na+ content under salt stress (Table 2). This suggests that Na+ was efficiently sequestred in vaccuoles during salt stress treatments. The proposed scenario to describe this strategy in that Na+ is pumped into vaccuole to prevent cytosolic Na+ toxicity (Blumwald et al. 2000, Shi et al. 2003) ; and even if some K+ may leak from the cytosol, it is quickly replenished from vacuole.
Table 2. Nodules number, nodule dry weight and biomass production (Shoot, Root length and dry weight) of pea-rhizobia combinations grown under optimal conditions and salt stress.
Treatments
Shoot P content
(mg/kg DW)
Shoot K content
(mg/kg DW)
Shoot Na content
(mg/kg DW)
Root P content
(mg/kg DW)
Root K Content
(mg/kg DW)
Root Na
content
(mg/kg DW)
Control
0.9±0.00
33.26±0.00
3.256±0.267
1.21±0.245
18.16±2.40
26.24±0.011
Ar02
1.09±0.07
33.89±0.02
3.324±0.013
1.22±0.140
18.32±1.23
28.46±0.636
Ar02+100mM
1.32±0.00
36.37±0.08
6.94±0.510
1.23±0.00
18.49±0.11
41.20±0.435
Data are means and SE of five replicates harvested at flowering stage.
Under salt stress, leaves H2O2 content significantly increased in the combinations of P-vulgaris and rhizobia strain (Fig.2). Worth to mention that highest level of H2O2 content was detected at flowering stage that was in order to 31µmol.g-1 FM in the symbiotic combination under 100mM NaCl. Concerning the H2O2 content in leaves, significant differences were noted in regard to control treatment (Figure 2).
|
Figure 2: H2O2 content in leaves of Common bean- rhizobia combinations grown under salt stress (100 mM). Data are means of four replicates harvested at flowering stage of culture. |
-
Peroxidase and polyphenol oxidase activities
Concerning the activity of PO in leaves, results showed a significant increase. PO was in order to 1.2 µmol g-1FM under salt stress. However, this activity was in order to 0.3 µmol g-1FM in controlled treatment. In general, salt stress affected slightly PO activity (Figure 3). The same for PPO activity that did not increased and was not exceed 10 µmol g-1FM . Compared to PO, salt stress slightly stimulated PPO activity in leaves (Figure 4). In this aspect, the observation of present study are contradictory with Aroca et al. (2003); Shanker et al. (2004) and Mandhania et al. (2006) those who reported in leaves of plant a significant variation of PO as an evidence of oxidative damage comparatively to control.
|
Figure 3: PO content in leaves of Common bean- rhizobia combinations grown under salt stress (100 mM). Data are means of four replicates harvested at flowering stage of culture.
|
Figure 4: PPO content in leaves of Common bean- rhizobia combinations grown under salt stress (100 mM). Data are means of four replicates harvested at flowering stage of culture. |
-
Effect on proline contents
The results indicated that salinity induced a significant increase in proline accumulation in leaves of common bean-rhizobia symbiosis (Figure 5). A higher accumulation of proline in leaves under salt stress (100mM NaCl) was in order to 0. 3 µM. 100mg FM-1. The proline contents in control root were 0.1µM. mg FM-1. Ghoulam et al. (2002) observed that the salt tolerance of Beta vulgaris L. varieties is closely related with the accumulation of proline in leaves. The proline may act not only as an osmolyte, but it may also help the cells to overcome oxidative stress in salt stressed plants (Rajendrakumar et al. 1994). Also, it has been demonstrated that salt tolerance in plant such as alfalfa ecotypes was associated with proline accumulation (Monirifar and Barghi 2009; Farissi et al. 2011).
|
Figure 5: Proline amount (µM.100 mg-1 FM) of the leaves, roots of Common bean-rhizobia symbiosis under salt stress (100 mM NaCl). Data are means and SE of four replicates at flowering stage. |
-
Total phenol content
Roots of salt stress symbioses P-vulgaris increased significantly their total phenol content (Figure 6). Extent increase was noted under salt stress treatment that show the highest values of phenol content (155 mg.g-1 FM). After inoculation, combination was shown the increase roots phenol contents. In the whole, the total phenol content in the roots did show a significant difference between control and salt stress treatment (Figure6). Zeyen et al. (1995) and Abdi et al (2012) reported that root phenols content was a type of resistance of plants to a biotic stress. In the same idea, many studies have reported that phenols concentration has been found to be higher in tolerance stress than in stress sensitive plants (Ashraf and Harris 2004).
|
Figure 6: Phenols content in roots of Common bean- rhizobia combinations grown under salt stress (100mM). Data are means of four replicates harvested at flowering stage of culture. |
-
Conclusion
In conclusion, the results of this study indicate that common bean inoculated with Ar02 demonstrated a variation in tolerance to NaCl concentration (100 mM NaCl). Of various antioxidant enzymes and metabolites activity such as PPO, H2O2 and proline content as well as the amounts of total antioxydants were found to be associated with the differential response of common bean-rhizobia symbiosis to salt stress. Moreover, despite the fact that the leaves are the organ of antioxidant activity analysis, the enzymatic reaction varies differently against salt stress. Significant variation exists between the redox states after inoculation in the leaves, which can help prevent the toxic accumulation of variability in the plant host under conditions of contrast over time.
Acknowledgements
This work was financially supported by the ministry of agriculture and the ministry of higher education and scientific research in Tunisia. We thank Dr. Adnane BARGAZ for the advice and help.
-
References
Abdi N, Bargaz A, Bouraoui M, Ltaief B, Ghoulam C , Sifi B (2012) Symbiotic responses to insoluble phosphorus supply in common bean (Phaseolus vulgaris L.): Rhizobia symbiosis. Afr. Jour Biotech 11(19): 4360-4367
Aroca R, Irigoyen JJ, Sanchez-Diaz M (2003) Drought enhances maize chilling tolerance. II. Photosynthetic traits and protective mechanisms against oxidative stress. Plant Physiol 117:540-549
Ashraf M , Harris PJC (2004) Potential biochemical indicators of salinity tolerance in plants. Plant Sci 166: 3-16
Bargaz A, Faghire M, Farissi M, Drevon JJ, Ghoulam C (2013) Oxidative stress in the root nodules of Phaseolus vulgaris is induced under conditions of phosphorus deficiency. Acta Physiol Plant 35:1633-1644
Bates L, Waldren RP, Teare JD (1973) Rapid determination of free proline for water stress studies. Plant Soil 39:205-207
Cassman KG, Whitney AS, Fox R L (1981) Phosphorous requirements of soybean and cowpea as affected by mode of N nutrition. Agronomie 73: 17-22
CIAT (Centre International Agriculture Tropical) 1992. Constraints and opportunities for improving bean production. A planning document 1993-98 and an achieving document 1987-92. Cali, Colombia: CIAT.
Chen Z, Pottosin II, Cuin TA, Fuglsang AT, Tester M, Jha D, Zepeda-Jazo I, Zhou M, Palmgren MG, Newman IA, Shabala S (2007) Root plasma membrane transporters controlling K+ /Na+ homeostasis in salt-stressed barley. Plant Physiol 145 (4):1714-1725
Cordovilla M P, Ocan A, Ligero F, Lluch C (1996) Growth and symbiotic performance of faba bean inoculated with Rhizobium leguminosarum biovar. viciae strains tolerant to salt. Soil Sci and Plant Nutr 42: 133-140
Cuin TA, Shabala S (2007) Compatible solutes reduce ROS-induced potassium efflux in Arabidopsis roots. Plant, Cell & Envir 30 (7):875-885
Diani Z, Ouarraki EL, Aissam S, Hsissou D , El Modafar C (2009) Induction of early oxidative events in soft wheat leaves inoculated with Septoria tritici and their relationship to resistance of Moroccan cultivars. Inter Jour Agri and Biol 11:351-359
Dicko MH, Hilhorst R, Gruppen H, Traore AS, Laane C (2002) Comparison of content in phenolic compounds, polyphenol oxides and peroxidase in grains of fifty sorghum varieties from Burkina Faso. Jour Agri Food Chem 50: 3780-3788
Farissi M, Bouizgaren A, Faghire M, Bargaz A , Ghoulam C (2011) Agro-physiological responses of Moroccan alfalfa (Medicago sativa L.) populations to salt stress during germination and early seedling stages. Seed Sci Tech 39: 389-401
Ghoulam C, Foursy A , Fares K (2002) Effect of salt stress on growth, inorganic ions and proline accumulation in relation to osmotic adjustment in sugar beet (Beta vulgaris L.). Envir and Exp Bot 47:39-50
Hori K, Wada A, Shibuta T (1997) Changes in phenoloxidase activities of the galls on leaves of Ulmus vidana formed by Tetraneura funiformis. Appl Entomol Zool 32:365-371
Kaous S, Dabes A, Slatni T, Labidi N, Drevon JJ , Abdelly C (2009) Root proliferation, proton efflux, and acid phosphatase activity in common bean (Phaseolus vulgaris) under phosphorus shortage. Plant Biol 52: 395-402
Krouma A, Ben Hamed K, Abdelly C (2008) Symbiotic response of common bean (Phaseolus vulgaris L.) to iron deficiency. Acta Physiol Plant 30:27-34
Logan B A (2005) Reactive oxygen species and photosynthesis.In: Smirnoff N, editor Antioxidants and reactive oxygen species in plants. Oxford: Blackwell, p. 250-67
Mandhania S, Madan S, Sawhney V (2006) Antioxidant defense mechanism under salt stress in wheat seedlings. Biol Plant 50:227-231
Marschner H (1995) Mineral Nutrition of Higher Plants, 2nd ed. Academic Press Inc.,London
Munns R , Tester M (2008) Mechanisms of salinity tolerance. Ann Rev Plant Biol 59: 651-681
Munns R, James R A , Lauchli A (2006) Approaches to increasing the salt tolerance of wheat and other cereals. Jour Exp Bot 57:1025-43
Murphy J , Riley J P (1962) A modified single solution method for the determination of phosphate in natural waters. Acta Anal Chem 27:31-36
Noreen Z , Ashrafa M (2009) Assessment of variation in antioxidative defense system in salt-treated pea (Pisum sativum) cultivars and its putative use as salinity tolerance markers. Plant Physiol 166: 1764-1774
Rajendrakumar C S, Reddy B V, Reddy A R (1994) Proline- protein interaction: protection of structural and functional integrity of M4 lactate dyhydrogenase. Bioch and Biophy Res Com 201: 957-963
Sairam RK, Srivastava GC (2002) Changes in antioxidant activity in subcellular fraction of tolerant and susceptible wheat genotypes in response to long term salt stress. Plant Sci 162:897-904
Shabala S, Demidchik V, Shabala L, Cuin TA, Smith SJ, Miller AJ, Davies JM, Newman IA (2006) Extracellular Ca2+ ameliorates NaCl- induced K+ loss from Arabidopsis root and leaf cells by controlling plasma membrane K+ permeable channels. Plant Physiol 141:1653-1665
Shanker AK, Djanaguiraman M, Sudhagar R, Chandrashekar CN, Pathmanabhan G (2004) Differential antioxidative response of ascorbate glutathione pathway enzymes and metabolites to chromium speciation stress in green gram (Vigna radiata (L.) R.Wilczek. cv CO 4) roots. Plant Sci 166:1035-1043
Shi H, Lee B H, Wu S J, Zhu JK (2003) Overexpression of a plasma membrane Na1/H1 anti porter gene improves salt tolerance in Arabidopsis thaliana. Nat Biotech 21: 81-85
Subbarao GV, Johansen C (1994) Strategies and scope for improving salinity tolerance in crop plants. In: Pessarakli M, editor. Handbook of plant and crop stress. New York: Marcel Dekker, p. 1069-87
Tejera NA , Campos R, Sanjuan J , Lluch C (2004) Nitrogenase and antioxidant enzyme activities in Phaseolus vulgaris nodules formed by Rhizobium tropici isogenic strains with varying tolerance to salt stress. Plant Physiol 161:329- 338
VelikovaV, Yordanov I , Adreva A ( 2000) Oxidative stress and some antioxidant systems in acid rain treated bean some antioxidant systems in acid rain treated bean plants; protective role of exogenous polyamines. Plant Sci 151:59-66
Vincent JM (1970) A Manual for the Practical Study of Root-Nodule Bacteria. Blackwell Scientific, Oxford
Zeyen RJ, Bushnell WR, Carver TLW, Robbins MP, Clark TA, Boyles DA, Vance CP (1995) Inhibiting phenylalanine ammonia lyase and cinnamyl-alcohol dehydrogenase suppresses Mla1 (HR) but not mlo5 (non-HR) barley powdery mildew resistances. Physiol Mol Plant Pathol 47: 119-140