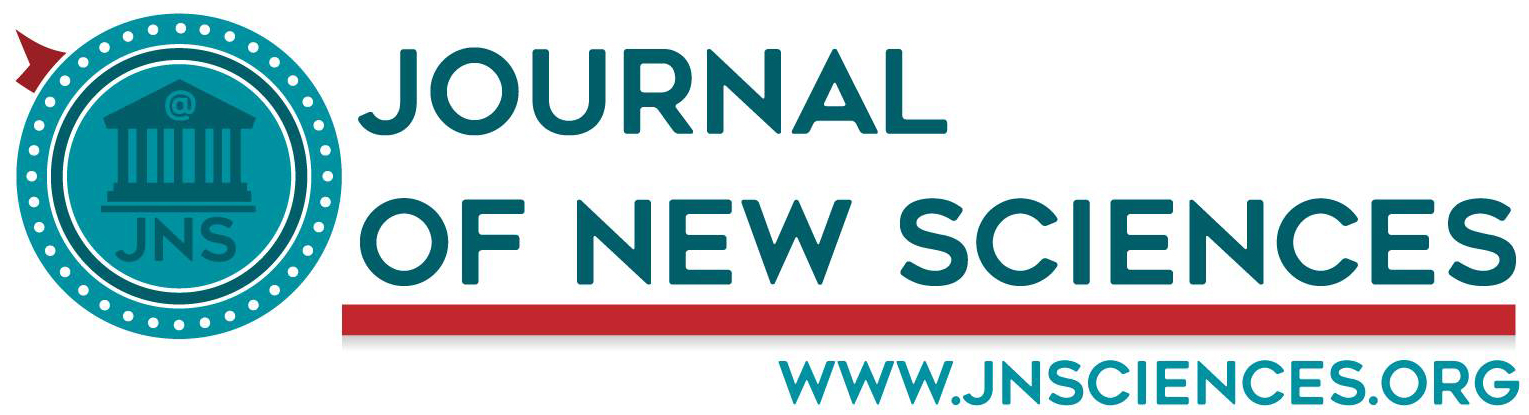
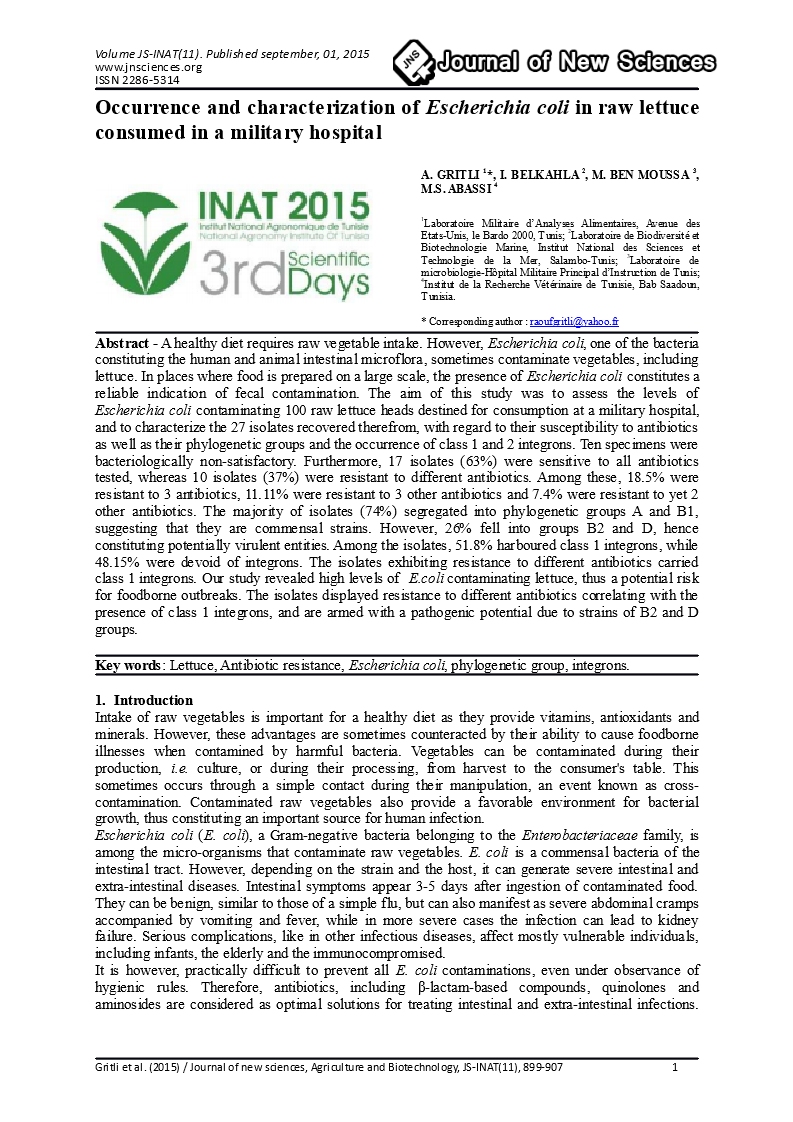
- Category: Volume Spécial (Journées Scientifiques de l'INAT)
- Hits: 5906
Occurrence and characterization of Escherichia coli in raw lettuce consumed in a military hospital
A. Gritli 1*
I. Belkahla 2
M. Ben Moussa 3
M.S. Abassi 4
1Laboratoire Militaire d’Analyses Alimentaires, Avenue des Etats-Unis, le Bardo 2000, Tunis; 2Laboratoire de Biodiversité et Biotechnologie Marine, Institut National des Sciences et Technologie de la Mer, Salambo-Tunis; 3Laboratoire de microbiologie-Hôpital Militaire Principal d’Instruction de Tunis; 4Institut de la Recherche Vétérinaire de Tunisie, Bab Saadoun, Tunisia.
Abstract - A healthy diet requires raw vegetable intake. However, Escherichia coli, one of the bacteria constituting the human and animal intestinal microflora, sometimes contaminate vegetables, including lettuce. In places where food is prepared on a large scale, the presence of Escherichia coli constitutes a reliable indication of fecal contamination. The aim of this study was to assess the levels of Escherichia coli contaminating 100 raw lettuce heads destined for consumption at a military hospital, and to characterize the 27 isolates recovered therefrom, with regard to their susceptibility to antibiotics as well as their phylogenetic groups and the occurrence of class 1 and 2 integrons. Ten specimens were bacteriologically non-satisfactory. Furthermore, 17 isolates (63%) were sensitive to all antibiotics tested, whereas 10 isolates (37%) were resistant to different antibiotics. Among these, 18.5% were resistant to 3 antibiotics, 11.11% were resistant to 3 other antibiotics and 7.4% were resistant to yet 2 other antibiotics. The majority of isolates (74%) segregated into phylogenetic groups A and B1, suggesting that they are commensal strains. However, 26% fell into groups B2 and D, hence constituting potentially virulent entities. Among the isolates, 51.8% harboured class 1 integrons, while 48.15% were devoid of integrons. The isolates exhibiting resistance to different antibiotics carried class 1 integrons. Our study revealed high levels of E.coli contaminating lettuce, thus a potential risk for foodborne outbreaks. The isolates displayed resistance to different antibiotics correlating with the presence of class 1 integrons, and are armed with a pathogenic potential due to strains of B2 and D groups.
Key words: Lettuce, Antibiotic resistance, Escherichia coli, phylogenetic group, integrons.
1. Introduction
Intake of raw vegetables is important for a healthy diet as they provide vitamins, antioxidants and minerals. However, these advantages are sometimes counteracted by their ability to cause foodborne illnesses when contamined by harmful bacteria. Vegetables can be contaminated during their production, i.e. culture, or during their processing, from harvest to the consumer's table. This sometimes occurs through a simple contact during their manipulation, an event known as cross-contamination. Contaminated raw vegetables also provide a favorable environment for bacterial growth, thus constituting an important source for human infection.
Escherichia coli (E. coli), a Gram-negative bacteria belonging to the Enterobacteriaceae family, is among the micro-organisms that contaminate raw vegetables. E. coli is a commensal bacteria of the intestinal tract. However, depending on the strain and the host, it can generate severe intestinal and extra-intestinal diseases. Intestinal symptoms appear 3-5 days after ingestion of contaminated food. They can be benign, similar to those of a simple flu, but can also manifest as severe abdominal cramps accompanied by vomiting and fever, while in more severe cases the infection can lead to kidney failure. Serious complications, like in other infectious diseases, affect mostly vulnerable individuals, including infants, the elderly and the immunocompromised.
It is however, practically difficult to prevent all E. coli contaminations, even under observance of hygienic rules. Therefore, antibiotics, including β-lactam-based compounds, quinolones and aminosides are considered as optimal solutions for treating intestinal and extra-intestinal infections. However, this decade has been marked by the emergence and dissemination of E. coli strains that are resistant to antibiotics, thus compromising treatment. Antibiotic resistance, a serious threat to public health safety, is carried by mobile genetic elements, such as integrons and plasmids.
Development of more sophisticated bacteriological technologies, combined with the use of molecular biology in clinical microbiology laboratories, enable a clear improvement in identification of pathogens, the number of which is alarmingly increasing, and provide crucial information useful for control of infections.
In this context, our present studies aimed at determining the extent of E. coli contamination of raw vegetables (lettuce heads) consumed in conjunction with large-scale food preparation in the Military Hospital of Tunis, as well as providing a phenotypic and a genotypic characterization of the bacteria. Our investigations enabed us to assess antibiotic susceptibility of a collection of 27 E. coli isolates from contaminated raw lettuce heads, identify their phylogenetic groups and determine the ocurrence of class 1 and 2 integrons.
2. Materials and Methods
Samples were recovered from 100 fresh lettuce heads in the kitchen of the Military Hospital in Tunis between April and July in 2013. Samples were taken out aseptically by a professional in food hygiene and kept cool during transfer to our laboratory. Upon arrival, undamaged fresh specimens were recorded before being processed. The samples were prepared according to the ISO 16649-1: 2001 standard.
2.2 Antibiotic susceptibility testings
Generation of an antibiogram enables in vitro determination of bacterial sensitivity to antibiotics. We generated antibiograms by using the agar diffusion method (Mueller-Hinton agar; Biokar Diagnostics) according to recommendations of Le Comité d’Antibiogramme de la Société Française de Microbiologie (CA-SFM, 2013).
Table 1. Distribution of E. coli phylogenetic groups and subgroups based on the presence (+) or absence (-) of specific DNA bands after gel electrophoresis. Adapted from Clermont et al. (2000) and Escobar-Paramo et al. (2006). |
||||
Chu A |
Yja A |
Tsp E4C2 |
Phylogenetic groups |
Subgroups |
(279bp) |
(211bp) |
(152bp) |
||
– |
– |
– |
A |
A0 |
– |
+ |
– |
A |
A1 |
+ |
+ |
– |
B2 |
B22 |
+ |
+ |
+ |
B2 |
B23 |
+ |
– |
– |
D |
D1 |
+ |
– |
+ |
D |
D2 |
– |
(±) |
+ |
B1 |
B1 |
2.3 Molecular characterization of E. coli isolates
We used the Polymerase Chain Reaction (PCR) assay to determine the phylogenetic groups and their distribution as well as the presence or absence of class 1 and 2 integrons in our lettuce-derived isolates.
2.3.1 Determination of the phylogenetic groups by PCR
E. coli strains segregate into four main phylogenetic groups (A, B1, B2, and D). Categorization of E. coli strains into a specific group is efficiently done by PCR which enables visualization of the presence or absence in their genome of three molecular markers, including the chuA and the yjaA genes as well as the DNA fragment TspE4C2 (Table 1), as demonstrated in previous studies (Clermont et al., 2000; Escobar-Paramo et al., 2006). The primers used to detect these genes (Clermont et al., 2000) are shown in Table 2.
Table 2. Primer sets and amplified fragments for identification of E. coli phylogenetic groups |
||
Locus |
Primers |
Size |
ChuA |
ChuA.1: 5’-GAC GAA CCA ACG GTC AGG AT-3’ |
279 bp |
ChuA.2: 5’-TGC CGC CAG TAC CAA AGA CA-3’ |
||
YjaA |
YjaA.1 : 5’-TGA AGT GTC AGG AGA CGC TG-3’ |
211 bp |
YjaA.2 : 5’-ATG GAG AAT GCG TTC CTC AAC-3’ |
||
TspE4C2 |
TspE4C2.1: 5’-GAG TAA TGT CGG GGC ATT CA-3’ |
152 bp |
TspE4C2.2: 5’-CGC GCC AAC AAA GTA TTA CG-3’ |
2.3.2 Detection and characterization of class 1 and 2 integrons
The presence or absence of class 1 and 2 integrons were determined using PCR. Specifically, detection of the intI1 and IntI2 genes encoding integrases of class 1 and class 2 integrons, respectively, was assayed by using specific primers (Table 3) as previously described (Mazel et al., 2010).
2.3.3 Agarose gel electrophoresis
PCR-amplified fragments were analysed by electrophoresis in agarose gels (2% in 0.5 x TBE buffer) containing 0.5 µl/ml ethidium bromide. The PCR products (15 µl) were mixed with 5 µl of TBE buffer shortly before loading into the gels. DNA bands were visualized and photographed under UV illumination.
Table 3. Primer sets and integron amplified fragments |
|
Genes/primer sequence (5’→3’) |
Size |
IntI 1 (class 1 integrase) |
|
GGGTCAAGGATCTGGATTTCG |
483 bp |
ACATGGGTGTAAATCATCGTC |
|
IntI 2 (class 2 integrase) |
|
CACGGATATGCGACAAAAAGGT |
788 bp |
GTAGCAAACGAGTGACGAAATG |
3.1 Prevalence of E. Coli in lettuce
We used the microbiological criteria implemented in the case of vegetable products according to the French ministerial rules and guidelines from May 28, 1997. These concern criteria applicable to raw, peeled and sliced vegetables as well as to ready-to-eat raw vegetables. Using these guidelines, we found that among 100 specimens analysed, 80% were of satisfactory quality, whereas 10% were acceptable for consumption and the remaining 10% were of unacceptable/non-satisfactory microbiological quality. This percentage of E. coli contamination is quite high as compared to findings by others. Indeed, Johannessen et al. (2002) assessed the bacteriological quality and potential health risk of 890 fresh and pre-sliced lettuce heads from Norwegian vegetable markets. The authors found the prevalence of thermo-tolerant coliforms to be 2.5%. Similarly, Meldrum et al. (2009), showed that among 1213 lettuce and vegetable specimens, 4.7% are of non-satisfactory bacteriological quality due to the presence of E. coli, with cucumbers being the most contaminated (6%).
However, in a study carried out from September 1999 to Mars 2000, Soriano et al. (2001) analysed 370 specimens from 20 restaurants in Valencia (Spain). The authors compared batches of unwashed lettuce with others that were pre-washed in water containing sodium hypochlorite. They found high prevalence (10%) of E. coli in unwashed specimens. The authors' findings concur with earlier studies by others (King et al., 1991) who found low prevalence of E. coli in washed/disinfected lettuce as compared to unwashed specimens. Thus, E. coli contamination could be used as a reliable indication of contamination during bacteriological assessments of crude products.
3.2 Antibiotic susceptibitity testings reveal resistance to several antibiotics
We carried out phenotypic tests for antibiotic resistance of 27 randomly selected E. coli isolates. We found that 17 isolates (63%) were sensitive to all antibiotics tested. Importantly however, we found that 10 isolates (37%) were resistant to antibiotics used in treatments of E. coli infections.
|
Figure 1. Distribution (percentages) of resistance of 27 E. coli isolates to each antibiotic |
Specifically, for each antibiotic tested (Fig. 1), none of the isolates studied were resistant to cefotaxime (CTX), piperacillin (PIP), ceftazidime (CAZ; a third-generation cephalosporin), ciprofloxacin (CIP), fosfomycin (FOS), kanamycin (K) and to the combination of Ticarcillin and clavulanic acid (TCC). The resistant isolates exhibited different profiles as follows: First, five isolates (18.5%) were resistant to three antibiotics, namely Amoxicillin without clavulanic acid (AMX), Amoxillin with clavulanic acid (AMC) and Ticarcillin (TIC). Second, three isolates (11.11%) were resistant to each of three other antibiotics, including Tetracyclines (Te), Nalidixic acid (NA) and Trimethoprim/Sulfamethoxazole (SXT). These latter 3 isolates exhibited hyperproduction of cephalo-sporinases. Finally, two isolates (7.4%) were found to be resistant to each of yet two other different antibiotics, including Chloramphenicol (C) and Gentamicin GM).
The entire collection of our isolates was unable to produce extended-spectrum β-lactamases. Resistance to amoxicillin is usually attributed to production of low- or high-level penicillinase (Caroff et al., 2000; Jorgensen et al., 2010).
Three (≈ 11%) of our isolates were resistant towards nalidixic acid, a quinolone. Resistance to quinolones is generally caused by mutations in specific regions of the genes encoding DNA gyrase A or topoisomerase IV. However, recent evidence points to the emergence of involvement of transmissible plasmid-mediated resistance to quinolones (Strahilevitz et al., 2009).
The nalidixic acid-resistant 3 isolates were also resistant to tetracyclines as well as to the combination Trimethoprim/Sulfamethoxazole. This low percentage (≈ 11%) is at odds with the high percentages of E. coli detected in human and animal sources in Tunisia and worldwide (Van et al., 2007; Meyer et al., 2008; Soufi et al., 2009). Nevertheless, tetracycline resistance in our isolates is alarming in a developing country, as it may reflect contamination of raw vegetables through contaminated irrigation water and manure.
We observed resistance to chloramphenicol and gentamicin only in two isolates (7.4%). These findings are in agreement with previous studies (Jouini et al., 2007; Soufi et al., 2009, 2011) showing low levels of resistance to these antibiotics.
|
Figure 2. Representative image (gel 1) of PCR profiles for the phylogenetic groups of E. coli isolates. L, 100 bp DNA ladder. Lanes 1, 2, 3, and 10, group A (Ao). Lanes 4, 5 and 9, group A (A1). Lanes 7 and 8, group B1. Lane 6, group D (D1). |
|
Figure 3. Representative image (gel 2) of PCR profiles for the phylogenetic groups of E. coli isolates. L, 100 bp DNA ladder. Lanes 11, 15, 16, 17, 19 and 21 portray group A (A0). Lane 18, group A (A1). Lane 22, group B1. Lanes 13 and 14, group B2 (B23). Lanes 12 and 20, group D (D1). |
3.3 Predominance of E.coli phylogenetic groups A and B1 in the isolate collection
For further characterization of the 27 E. coli isolates selected from raw lettuce, we carried out assays to determine their phylogenetic groups and subgroups. Representative images of the analyses of PCR products carried out to determine the distibution of the phylogenetic groups and subgroups (presence or absence of the genes ChuA, YjaA and TspE4C2) in E. coli isolates are shown in Figures 2 and 3.
We found that 16 isolates (59.3%) belonged to group A, among these 12 (44.5%) and 4 (14.8%) isolates were of the subgroups Ao and A1, respectiveley. Four isolates (14.8%) segregated into group/subgroup B1. Two isolates (7.4%) fell into group B2, none (0%) of which were of subgroup B22, whereas the two segregated into subgroup B23. Finally, 5 isolates (18.5%) belonged to group D, among these 4 isolates (14.8%) segregated in subgroup D1 and 1 isolate (3.7%) was categorized as subgroup D2. The distribution of the phylogenetic groups of the E. coli isolates is summarized in Table 4.
From our data, it emerges that E. coli strains of the phylogenetic groups A and B1 were predominant as they represented 74% (20 isolates) of our isolates, whereas groups B2 and D were under-represented since only 7 isolates (26%) were assigned to these groups. Overall, our findings echo those described in previous studies where groups A, B1 and D were found to be more prominent than group B2 (Ho et al., 2009). It is well-established that E. coli strains belonging to the B2 group are the most virulent, followed by group D strains, contrasting with those of group A and B1, which are commensal strains (Bonacorsi et al., 2005; Ewerset et al., 2007). Hence, we can conclude that 26% of our isolates are potentially virulent.
Table 4. Distribution of E. coli phylogenetic groups and subgroups among 27 isolates |
|||||
Isolate |
ChuA |
YjaA |
TspE4C2 |
Phylogenetic group |
Subgroup |
N° |
(279pb) |
(211pb) |
(152pb) |
||
1 |
– |
– |
– |
A |
A0 |
2 |
– |
– |
– |
A |
A0 |
3 |
– |
– |
– |
A |
A0 |
4 |
– |
+ |
– |
A |
A1 |
5 |
– |
+ |
– |
A |
A1 |
6 |
+ |
– |
– |
D |
D1 |
7 |
– |
– |
+ |
B1 |
B1 |
8 |
– |
– |
+ |
B1 |
B1 |
9 |
– |
+ |
– |
A |
A1 |
10 |
– |
– |
– |
A |
A0 |
11 |
– |
– |
– |
A |
A0 |
12 |
+ |
– |
– |
D |
D1 |
13 |
+ |
+ |
+ |
B2 |
B23 |
14 |
+ |
+ |
+ |
B2 |
B23 |
15 |
– |
– |
– |
A |
A0 |
16 |
– |
– |
– |
A |
A0 |
17 |
– |
– |
– |
A |
A0 |
18 |
– |
+ |
– |
A |
A1 |
19 |
– |
– |
– |
A |
A0 |
20 |
+ |
– |
– |
D |
D1 |
21 |
– |
– |
– |
A |
A0 |
22 |
– |
– |
+ |
B1 |
B1 |
23 |
– |
– |
– |
A |
A0 |
24 |
+ |
– |
+ |
D |
D2 |
25 |
– |
– |
+ |
B1 |
B1 |
26 |
– |
– |
– |
A |
A0 |
27 |
+ |
– |
– |
D |
D1 |
3.4. Positive correlation between the presence of integrons and resistance to antibiotics
It is well-established that bacterial resistance to antibiotics is a major problem in human medicine as it thwarts the treatment of infectious diseases. This is even further compounded by the increasing emergence of bacterial strains exhibiting multi-drug-resistance (Hue et al., 2010). Dissemination of antibiotic resistance in Enterobacteriaceae is generally due to expression of resistance genes located on integrons or transposons, which can be chromosomal or plasmidic. Integrons provide a genetic basis for capture and expression of resistance genes as they can harbour 1 to 8 resistance genes (Gillings et al., 2008).
|
Figure 4. Representative image of PCR profiles specific to the IntI 1 and IntI 2 genes. L, 100 bp ladder. C, positive control isolate showing the presence of class 1 and class 2 integrons. Lanes 2, 4, 5, 7, 8, 10 and 11, isolates with class 1 integron. Lanes 1, 3, 6 and 9, isolates free of integrons. |
Among the 27 isolates studied, PCR assays (representative data of these experiments are shown in Fig. 4) indicated that 14 isolates (51.8%) harbour class 1 integron, whereas class 2 integron was not detected. This emphasizes the predominance of class 1 integrons over that of class 2. Furthermore, we found that 13 isolates (48.15%) were devoid of integrons. Our findings are similar to those of others (Soufi et al., 2009, 2011; Domingues et al., 2012). In this regard, class 1 integrons are genetically active entities as they are able to capture and express gene cassettes encoding antibiotic resistance factors, whereas class 2 integrons are stable units and unable to integrate new gene cassettes.
By providing a global view of resistance to antibiotics combined with determination of the presence/absence of integrons in 27 isolates (Table 5), we were able to reveal a positive correlation between antibiotic resistance and presence of integrons. The ten E. Coli isolates (37%; isolates 2, 4, 5, 7, 8, 10, 11, 19, 20 and 27) that displayed resistance to different antibiotics all harboured class 1 integrons, further emphasizing the crucial role of integrons in dissemination and acquisition of resistance genes. Remarkably, 4 isolates were sensitive to antibiotics, yet carried class 1 integrons, suggesting the presence of a genetic pool for potential capture of resistance genes and a likelihood of presence of empty integrons, as shown in previous studies (Quiroga et al., 2013; Rowe-Magnus et al., 1999).
Table 5. Summary of the findings in the 27 E. coli isolates |
||||
Isolate |
Phylogenetic group |
Subgroups |
Resistance profile |
Integrons |
E. coli 1 |
A |
A0 |
Sensitive |
Absent |
E. coli 2 |
A |
A0 |
Resistant to (AMX), (TIC) and (AMC) |
Presence of class 1 integron |
E. coli 3 |
A |
A0 |
Sensitive |
Absent |
E. coli 4 |
A |
A1 |
Resistant to (AMX), (TIC) and (AMC) |
Presence of class 1 integron |
E. coli 5 |
A |
A1 |
Resistant to (AMX), (TIC) and (AMC) |
Presence of class 1 integron |
E. coli 6 |
D |
D1 |
Sensitive |
Absent |
E. coli 7 |
B1 |
B1 |
Resistant to (Te), (NA) and (SXT) |
Presence of class 1 integron |
E. coli 8 |
B1 |
B1 |
Resistant to (Te), (NA) and (SXT) |
Presence of class 1 integron |
E. coli 9 |
A |
A1 |
Sensitive |
Absent |
E. coli 10 |
A |
A0 |
Resistant to (C) and (GM) |
Presence of class 1 integron |
E. coli 11 |
A |
A0 |
Resistant to (Te), (NA) and (SXT) |
Presence of class 1 integron |
E. coli 12 |
D |
D1 |
Sensitive |
Absent |
E. coli 13 |
B2 |
B23 |
Sensitive |
Absent |
E. coli 14 |
B2 |
B23 |
Sensitive |
Absent |
E. coli 15 |
A |
A0 |
Sensitive |
Absent |
E. coli 16 |
A |
A0 |
Sensitive |
Absent |
E. coli 17 |
A |
A0 |
Sensitive |
Absent |
E. coli 18 |
A |
A1 |
Sensitive |
Absent |
E. coli 19 |
A |
A0 |
Resistant to (AMX), (TIC) and (AMC) |
Presence of class 1 integron |
E. coli 20 |
D |
D1 |
Resistant to (AMX), (TIC) and (AMC) |
Presence of class 1 integron |
E. coli 21 |
A |
A0 |
Sensitive |
Presence of class 1 integron |
E. coli 22 |
B1 |
B1 |
Sensitive |
Presence of class 1 integron |
E. coli 23 |
A |
A0 |
Sensitive |
Presence of class 1 integron |
E. coli 24 |
D |
D2 |
Sensitive |
Presence of class 1 integron |
E. coli 25 |
B1 |
B1 |
Sensitive |
Absent |
E. coli 26 |
A |
A0 |
Sensitive |
Absent |
E. coli 27 |
D |
D1 |
Resistant to (C) and (GM) |
Presence of class 1 integron |
4. Conclusion
The presence of E. coli in food products, and notably in raw vegetables, constitutes a potential risk for food illness outbreaks, especially in hospitals where food is prepared on a large scale. In this context, the most alarming is the fact that raw vegetables are served to vulnerable patients who are already weakened by other diseases, advanced age, or subsequent to various invasive surgeries. This indeed constitute a major public health issue.
E. coli is an endogenous, benign bacteria found normally among the intestinal microflora. However, pathogenic strains occasionally induce intestinal and extra-intestinal infections. E. coli belongs to the coliform group of thermo-tolerant bacteria, able to survive and grow at temperatures reaching 44 °C. Furthermore, E. coli is an excellent "reporter bacteria", indicating fecal contamination of raw vegetables.
We show that 10% of lettuce heads analysed were contaminated. This level is relatively high when compared to previous findings by others. Despite low levels of resistance to antibiotics in our E. coli isolates, it would be necessary to establish a program to control antibiotic resistance in laboratories in Tunisia. Resistance to different antibiotics was of 37%. The distribution of resistance among these includes, 18.5% towards AMX, TIC and AMC, 11.11% to Te, NA and SXT, as well as 7.4% to C and GM.
The majority of our isolates (74%) segregated into the phylogenetic groups A and B1, known to include commensal strains. However, this result does not totally exclude pathogenicity of these isolates.
The predominant existence of class 1 integrons detected in 51.8% of the isolates over that of class 2 integrons, which was not detected, suggests that these are endowed with a powerful genetic basis for capture and expression of resistant genes, entailing further resistance gene dissemination. The 37% isolates that were resistant to different antibiotics, all contained class 1 integrons, further strengthening the role of these genetic entities in the spread and acquisition of antibiotic resistance genes.
Our data showing the presence of relatively high levels of E. coli displaying a range of potential threatening characteristics, combined with the fact that the isolates were from raw lettuce consumed by patients and personnel in a hospital, emphasize the urgent need for the establishment and implementation of control programs, such as the Hazard Analysis & Critical Control Points (H.A.C.C.P), together with improving public awareness. These are necessary measures to prevent catastrophic outbreaks.
5. References
Bonacorsi, S., Bingen, E. (2005). Molecular epidemiology of Escherichia coli causing neonatal meningitis. Int J Med Microbiol 295: 373–38.
Caroff, N., Espaze, E., Gautreau, D.,Richet, H., Reynaud, A. (2000). Analysis of the effects of 242 and 232 ampC promoter mutations in clinical isolates of Escherichia coli hyperproducing AmpC. J Antimicrob Chemother 45: 783–788.
Clermont, O., bonacorsi, S., Bingen, E. (2000). Rapid and simple determination of the Escherichia coli phylogenetic group. Appl Env Microbiol 66: 4555-4558.
Domingues, S., Da Silva, G.J., Nielsen, K.M. (2012). Integrons: vehicles and pathways for horizontal dissemination in bacteria. Mob Genet Elements 2: 211-223.
Escobar-Paramo, P., Clermot, O., Blanc-Potard, A.B., Bui, H., Le Bouguenec, C., Denamur, E. (2006). A specific genetic background is required for acquisition and expression of virulence factors in Escherichia coli. Mol Biol Evol 21: 1085-1094.
Ewerset, C., Li, G., Wilking, H., Kiebling, S., Alt, K., Antao, E.M., Laturnus, C., Diehl I., Glodde, S., Homeier, T., Bohnke, U., Steinruck, H., Philipp, H.C., Wieler, L.H. (2007). Avian pathogenic, uropathogenic, and newborn meningitis-causing Escherichiacoli: How closely related are they. Int J Med Microb 297:163-176.
Gillings, M., Boucher, Y., Labbate, M., Holmes, A., Krishnan, S., Holley, M., Stokes, HW. (2008). The evolution of class 1 integrons and the rode of antibiotic resistance. J Bacteriol 190: 5095-5100.
Ho, P.L., Wong, R.C., Chow, K.H., Que, T.L.(2009). Distribution of integrin associated trimethoprim-sulfamethoxazole resistance determinants among Escherichia coli from humans and food-producing animals. Lett Appl Microbiol 49: 627-34.
Hur, J., Kim, JH., Park, JH., Lee, YJ., Lee, JH.(2010). Molecular and virulence characteristics of multi-drug resistant Salmonella Enteritidis strains isolated from poultry. Vet J 10: 7-17.
Johannessen, G.S., Loncarevic, S., Kruse, H. (2002). Bacteriological analysis of fresh produce in Norway. Int J Food Microbiol 77: 199-204.
Jouini, A., Vinué, L., Ben Slama, K., Sáenz, Y., Klibi, N., Hammami, S., Boudabous, A., Torres, C., (2007). Characterization of CTX-M and SHV extended-spectrum beta-lactamases and associated resistance genes in Escherichia coli strains of food samples in Tunisia. J Antimicrob Chemother 60: 1137-114.
Jorgensen, R.L., Nielsen, J.B., Friis-Møller, A., Fjeldsøe, N.H., Schønning, K. (2010). Prevalence and molecular characterization of clinical isolates of Escherichia coliexpressing an AmpC phenotype. J Antimicrob Chemother 65: 460–464.
King, Jr. A.D., Magnuson, J., Goodman, N. (1991). Microbial Flora and storage quality of partially processed lettuce. J Food Sci 56: 459-461.
Mazel, D., Dychinco, B., Webb, V.A., Davies, J. (2000). Antibiotic resistance in the ECOR collection: integrons and identification of a novel aad gene. Antimicrob Agents Chemother 44: 1568-1574.
Meldrum, R.J., Little, C.L., Sagoo, S., Mithani, V., M.C Lauchlin, J., De pinna E. (2009). Assessment of the microbiological safety of salad vegetables and sauces from kebab take-away restaurants in the United Kingdom. Food Microbiol 26: 573-577.
Meyer, E., Lunke, C., Kist, M., Schwab, F., Frank, U. (2008). Antimicrobial resistance in Escherichia coli strains isolated from food, animals and humans in Germany. Infection 36: 59-61.
Quiroga, M.P., Arduino, S.M., Merkier, A.K., Quiroga, C., Petroni, A., Argentinian Integron Study Group Paul H. Roy., Centron, D. (2013). Distribution and functional identification of complex class 1 integrin. Infect Genet Evol 19: 88-96.
Rowe-Magnus, D.A., Guerout, A.M., Mazel, D. (1999). Super-intégrons. Res Microbiol 150: 641-51.
Soriano, J.M., Rico, H., Molto, J.C., Manes, J. (2001). Incidence of microbial flora in lettuce, meat and Spanish potato omlette from restaurants. Food Microbiol 18:159-163.
Soufi, L., Abbassi, M.S., Sáenz, Y., Vinué, L., Somalo, S., Zarazaga, M., Abbas, A., Dbaya, R., Khanfir, L., Ben Hassen, A., Hammami, S, Torres C. (2009). Prevalence and diversity of integrons and associated resistance genes in Escherichia coli isolates from poultry meat in Tunisia. Foodborne Pathog Dis 6: 1067-1073.
Soufi, L., Sáenz, Y., Vinué, L., Abbassi, M.S., Ruiz, E., Zarazaga, M., Ben Hassen, A., Hammami, S., Torres, C. (2011). Escherichia coli of poultry food origin as reservoir of sulphonamide resistance genes and integrons. Int J Food Microbiol 144: 497-502.
Strahilevitz, J., Jacoby, G.A., Hooper, D.C., Robicsek, A. (2009). Plasmid-mediated quinolone resistance: a multifaceted threat. Clin Microbiol Rev 22: 664-689.
Van, T.T., Moutafis, G., Tran, L.T., Coloe, P.J. (2007). Antibiotic resistance in food-borne bacterial contaminants in Vietnam. Appl Environ Microbiol 73: 7906-7911.