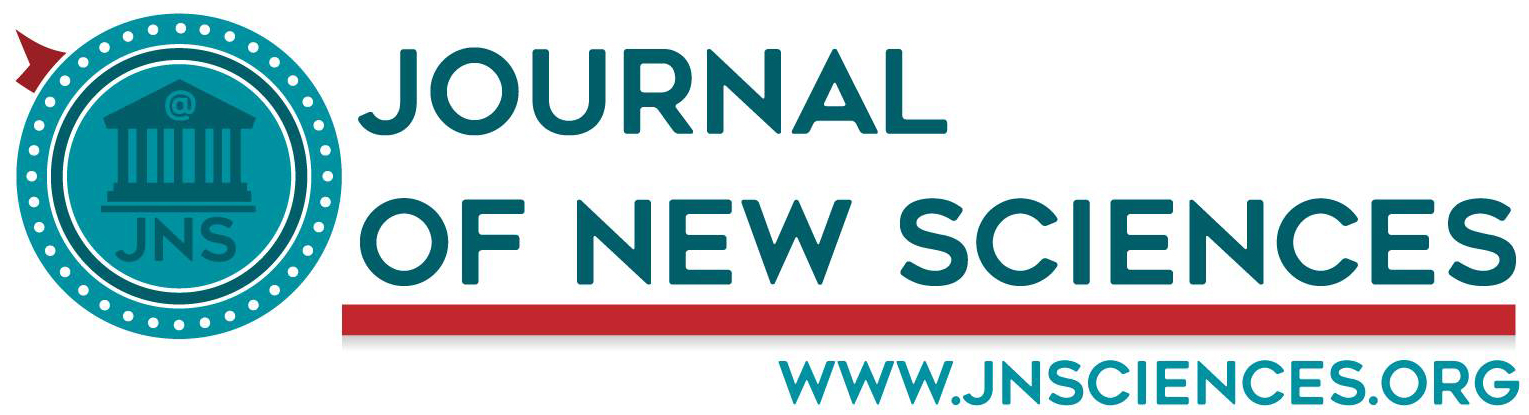
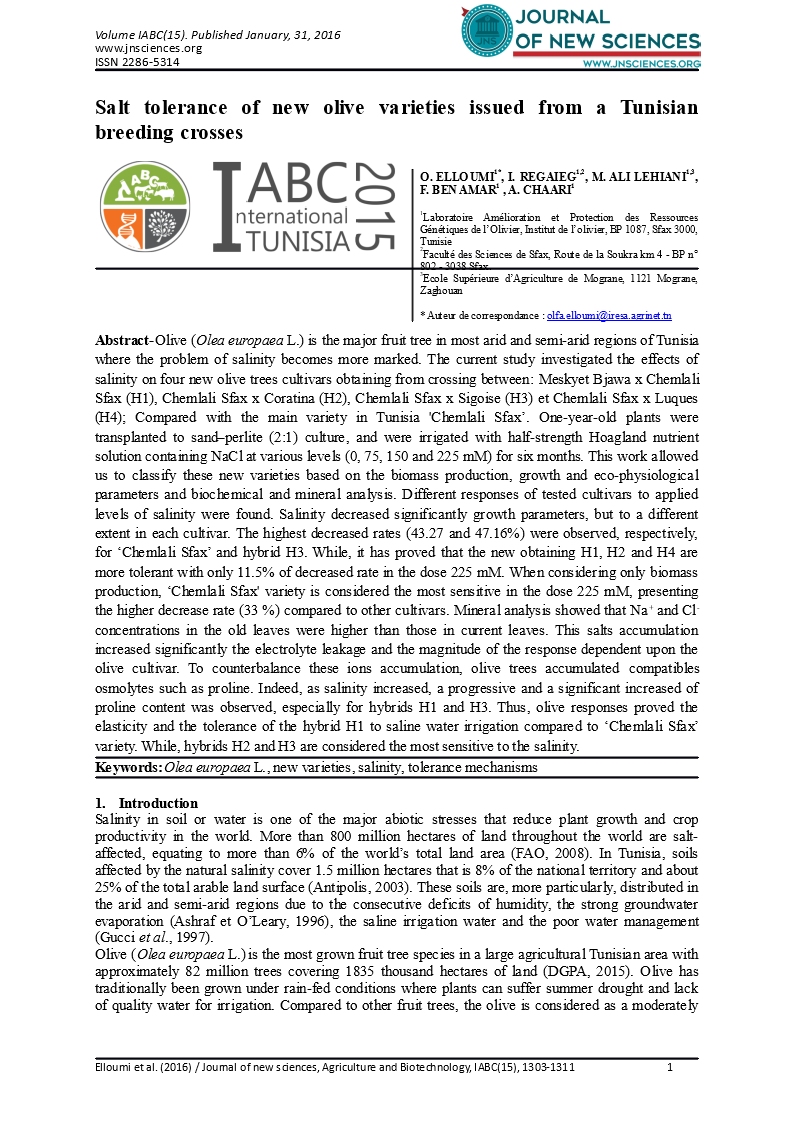
- Category: Volume Spécial (Conférence IABC 2015)
- Hits: 6377
Salt tolerance of new olive varieties issued from a Tunisian breeding crosses
O. Elloumi1*
I. Regaieg1,2
M. Ali Lehiani1,3
F. Ben Amar1
A. Chaari1
1Laboratoire Amélioration et Protection des Ressources Génétiques de l’Olivier, Institut de l’olivier, BP 1087, Sfax 3000, Tunisie
2Faculté des Sciences de Sfax, Route de la Soukra km 4 - BP n° 802 - 3038 Sfax.
3Ecole Supérieure d’Agriculture de Mograne, 1121 Mograne, Zaghouan
Abstract-Olive (Olea europaea L.) is the major fruit tree in most arid and semi-arid regions of Tunisia where the problem of salinity becomes more marked. The current study investigated the effects of salinity on four new olive trees cultivars obtaining from crossing between: Meskyet Bjawa x Chemlali Sfax (H1), Chemlali Sfax x Coratina (H2), Chemlali Sfax x Sigoise (H3) et Chemlali Sfax x Luques (H4); Compared with the main variety in Tunisia 'Chemlali Sfax’. One-year-old plants were transplanted to sand–perlite (2:1) culture, and were irrigated with half-strength Hoagland nutrient solution containing NaCl at various levels (0, 75, 150 and 225 mM) for six months. This work allowed us to classify these new varieties based on the biomass production, growth and eco-physiological parameters and biochemical and mineral analysis. Different responses of tested cultivars to applied levels of salinity were found. Salinity decreased significantly growth parameters, but to a different extent in each cultivar. The highest decreased rates (43.27 and 47.16%) were observed, respectively, for ‘Chemlali Sfax’ and hybrid H3. While, it has proved that the new obtaining H1, H2 and H4 are more tolerant with only 11.5% of decreased rate in the dose 225 mM. When considering only biomass production, ‘Chemlali Sfax' variety is considered the most sensitive in the dose 225 mM, presenting the higher decrease rate (33 %) compared to other cultivars. Mineral analysis showed that Na+ and Cl- concentrations in the old leaves were higher than those in current leaves. This salts accumulation increased significantly the electrolyte leakage and the magnitude of the response dependent upon the olive cultivar. To counterbalance these ions accumulation, olive trees accumulated compatibles osmolytes such as proline. Indeed, as salinity increased, a progressive and a significant increased of proline content was observed, especially for hybrids H1 and H3. Thus, olive responses proved the elasticity and the tolerance of the hybrid H1 to saline water irrigation compared to ‘Chemlali Sfax’ variety. While, hybrids H2 and H3 are considered the most sensitive to the salinity.
Keywords: Olea europaea L., new varieties, salinity, tolerance mechanisms
-
Introduction
Salinity in soil or water is one of the major abiotic stresses that reduce plant growth and crop productivity in the world. More than 800 million hectares of land throughout the world are salt-affected, equating to more than 6% of the world’s total land area (FAO, 2008). In Tunisia, soils affected by the natural salinity cover 1.5 million hectares that is 8% of the national territory and about 25% of the total arable land surface (Antipolis, 2003). These soils are, more particularly, distributed in the arid and semi-arid regions due to the consecutive deficits of humidity, the strong groundwater evaporation (Ashraf et O’Leary, 1996), the saline irrigation water and the poor water management (Gucci et al., 1997).
Olive (Olea europaea L.) is the most grown fruit tree species in a large agricultural Tunisian area with approximately 82 million trees covering 1835 thousand hectares of land (DGPA, 2015). Olive has traditionally been grown under rain-fed conditions where plants can suffer summer drought and lack of quality water for irrigation. Compared to other fruit trees, the olive is considered as a moderately salt tolerant plant (Rugini and Fedeli, 1990) and the tolerance level is cultivar and plant-age dependent (Chartzoulakhis, 2005; Tattini et al., 1992). Salt tolerance in olive cultivars is associated with effective mechanisms of ion exclusion and retention of Na+ and Cl− in the root (Chartzoulakhis et al., 2002), limiting the accumulation of these ions into actively growing shoots. When olive is irrigated with water containing NaCl, growth reduction and onset of damage are more correlated to Na+ than Cl- accumulation in leaves (Tattini et al., 1992; Perica et al., 2008). In salt stress condition, accumulation of K+ and compatible solutes will promote the maintaining of sub-cellular structure and cellular turgor and the increase in the osmotic potential of the cell in plants (Ashraf and Foolad, 2007).
Furthermore, cell membranes are one of the first targets of many plant stresses and it is generally accepted that the maintenance of their integrity and stability under water stress conditions is a major component of drought tolerance in plants. The degree of cell membrane injury induced by salt stress may be easily estimated through measurements of electrolyte leakage from the cells. Such a technique has been applied to quantify damages to cell membranes in seven olive cultivars growing under increased salinity (Perica et al., 2008). Results showed that electrolyte leakage was linearly increased with increasing salinity and the magnitude of the response depended upon the olive cultivar.
Many olive cultivars are present in Tunisia (Trigui et al., 2002), but the Tunisian olive orchards are dominated by the “Chemlali” cultivar population that is planted in almost all regions, covering 2/3 of the Tunisian olive grove and contributing more than 60% of the national production of the olive oil (Khlif et al., 2002; Ben Rouina et al., 2002). This cultivar is characterized by the strongest average production in the world and by it’s resistant to droughts. ‘Chemlali de Sfax’ olive tree is characterized by relatively low levels of oleic acid (53–60%), high levels of palmitic and a low oleic/linoleic acid ratio (Grati and Khlif et al., 2001). For this reason, a breeding crosses program was undertaken from 1990 to improve the oil quality of this cultivar (Trigui and Msallem et al., 2001) and to develop new varieties that can grow and produce economic yields under severs conditions.Thus, the aim of this work was to investigate and compare the effect of salinity on the biomass production, growth and eco-physiological parameters and biochemical and mineral analysis of four new olive trees cultivars issued from a Tunisian hybridization program grown under controlled conditions, in order to assess NaCl salinity tolerance and to determine the level of NaCl at which the plants start showing the salinity symptoms.
2.Materials and methods
2.1. Plant material and growth conditions
Four uniform one-year-old plants of new olive trees cultivars issued from a Tunisian breeding program were used in this work : Meskyet Bjawa x Chemlali Sfax (H1), Chemlali Sfax x Coratina (H2), Chemlali Sfax x Sigoise (H3); and were compared with the main variety in Tunisia ' Chemlali Sfax’. Before transplanting plants into pots of 8 l containing sand–perlite mixture (2:1), roots were washed with water to eliminate residues of substrate. Plants were irrigated daily with a half-strength Hoagland nutrient solution with the following composition (mM) (2.5 KNO3, 1 MgSO4, 1 KH2PO4, 2.5 Ca(NO3)_4H2O, and 0.5 NaCl) and (mM) (4.6 MnCl2, 23.1H3BO3, 0.06 Na2MoO4, 1.2 ZnSO4 and 0.19 CuSO4). Plants were grown in these conditions during two months. Salinity treatments were imposed after the two months period of adaptation. A control (0 mM) and three levels of salinity were used in this experiment. Control plants were irrigated with ½ Hoagland nutrient solution. For salinity treatments, NaCl was added to the ½ Hoagland nutrient solutions at 75, 150 and 225 mM. One hundred plants were used in this experiment corresponding to five plants per cultivar for each treatment.
2.2. Measured parameters
Growth measurements were carried out on five trees per treatment and by variety. The length of shoot (in cm) and the trunk section (in mm) were measured at the beginning and the end of the exposure to salinity. The experiment was terminated after six months. At the end of this period, three plants were gently removed from pots and separated into roots, shoot and leaves fractions. The dry weight (DW) of shoot, leaves and roots was determined after drying at 70 °C for 72h.
The mineral composition of old and current leaves (developed, respectively, before and after the beginning of exposure to salinity) was determined at the end of the experiment. Leaves were harvested, intensively washed with de-ionized water and analyzed for sodium (Na), chloride (Cl) and potassium (K). Sodium and potassium were determined by flame emission photometer (JENWAY), and chloride by the Mohr method. Results were expressed as percentage of dry weight (DW).
Approximately six-month old leaves, developed at the beginning of the salinity treatments were used for electrolyte leakage measurement by modified procedures described by Lutts et al. (1996). One leaf from three plants per replication was taken and cut into nine leaf discs of 1cm of diameter. Samples were washed three times with distillated water to remove surface-adhered electrolytes. Leaf discs were placed in closed vials containing 10 ml of distillated water and incubated at 25°C on a rotary shaker (100 rpm) for 24 h. Electrical conductivity of bathing solution (EC1) was recorded after incubation. The same samples were then autoclaved at 120ºC for 20 min and second reading (EC2) was taken after cooling the solution to room temperature. The electrolyte leakage was calculated as: EL (in %) = (EC1/EC2) x100.
Proline colorimetric determination proceeded according to Bates et al. (1973) based on proline's reaction with ninhydrin and using L-proline for the standard curve (0.01-0.15 mmol/l).
The relaive water content (RWC) was determined as following (Gucci et al., 1997). Five expanded leaves per treatment were collected from the median part of shoots. Leaves were weighted fresh (Fw) and placed in distilled water in the dark for 24 hours to rehydrate. The following morning, leaf turgid weight (Tw) was measured and then leaves were dried at 80°C for 48 hours and dry weight (Dw) was determined. RWC (%) = [(Fw – Dw) / (Tw – Dw)] x 100.
2.3. Data analysis
Statistical analyses were performed using the SPSS for Windows (Statistical Package of the Social Sciences) base 13.0 software (Chicago, IL, USA). The treatment means were compared using Duncan's test calculated at 5% level. At least three replicates were used for each laboratory test.
3. Results and discussion
-
Growth response and biomass allocation
Plant growth characterization was established by measuring the relative shoot growth, the trunk section and biomass allocation at the end of the experiment for all cultivars (table 1 and 2). Generally, all data published on olive shows that plant growth (shoot length, total leaf area, dry weight, root length and rooting ability) is inhibited by moderate and high salinity (Chartzoulaki, 2005; Kchaou et al., 2010; Bader et al., 2015) to varying degree. In the present study, results showed that up to 150 mM NaCl, shoot growth and trunk section were significantly affected in ‘Chemlali Sfax’ (table 1). However, no significant effect was observed for the new olive cultivars issued from breeding crosses, with the exception of H1 trunk section at 150 and 225 mM that was significantly reduced compared to the control.
Also, the effect of salinity on the biomass allocation showed a significant genotypic variation (table 2). Indeed, the highest reductions in shoot and root dry weights were found in ‘Chemlali Sfax’ cultivar which reached, respectively, by 89.5 and 59.7 % at 150 mM NaCl.
Total plant dry weight was significantly reduced in ‘Chemlali Sfax’ cultivar from the concentration of 150 mM NaCl, while from the concentration of 225 mM for hybrids H2 and H3. At 150 mM NaCl, the highest decrease of total plant dry weight was observed for ‘Chemlali Sfax’, which was about 33.3% with respect to the control plants. At 225 mM NaCl, total plant dry weight reduction was less severe (22.2%) for hybrids H2 and H3. The decrease in total plant dry weight could be ascribed essentially to the decrease of both the dry weight of the aerial part (principally the shoot mass) and roots. However, the dry weights of leaves were unaffected by salt treatments for all cultivars.
Balanced growth models assume that biomass is preferentially allocated to the plant part that obtains resources in growth limiting conditions such as the root system (Shipley and Meziane 2002). Analysis of biomass partitioning, in this study, showed a decreased allocation in the below ground plant parts as salinity increased. More precisely, the root portion related to the shoot weight decreased, in the case of ‘Chemlali Sfax’, H1 and H4 cultivars, as the salinity of the nutrient solution increased (table 2).
Table1. Effect of NaCl salinity on shoot growth and trunk diameter of five olive cultivars grown at four levels of NaCl (0, 75, 150 and 225 mM) for 180 days. |
|||
|
NaCl (mM) |
shoot growth (cm) |
Trunk diameter (mm) |
Chemlali Sfax |
0 |
67.33 b |
2.14 b |
75 |
42.57 ab |
1.54 ab |
|
150 |
40.07 ab |
1.35 ab |
|
225 |
38.20 a |
0.41 a |
|
|
|
|
|
H1 |
0 |
44.03 a |
2.20 b |
75 |
44.93 a |
1.52 ab |
|
150 |
54.30 a |
1.15 a |
|
225 |
38.93 a |
1.14 a |
|
|
|
|
|
H2 |
0 |
45.40 a |
1.54 a |
75 |
60.90 a |
1.31 a |
|
150 |
70.70 a |
1.16 a |
|
225 |
40.30 a |
1.26 a |
|
|
|
|
|
H3 |
0 |
54.07 a |
2.09 a |
75 |
47.75 a |
2.18 a |
|
150 |
111.17 b |
2.43 a |
|
225 |
28.57 a |
1.77 a |
|
|
|
|
|
H4 |
0 |
36.63 a |
1.26 a |
75 |
52.27 a |
1.36 a |
|
150 |
62.73 a |
1.26 a |
|
225 |
32.27 a |
1.08 a |
Means within the column followed by the same letter are not significantly different at p = 5% level, using Duncan’s test
Table2. Effect of NaCl salinity on shoot, leaf, root and total plant dry weight and root/aerial part ratio of five olive cultivars grown at four levels of NaCl (0, 75, 150 and 225 mM) for 180 days. |
||||||
|
NaCl (mM) |
Shoot dry weight (g) |
Leaf dry weight (g) |
Root dry weight (g) |
Total plant dry weight (g) |
Roots/Aerial part |
Chemlali Sfax |
0 |
7.49 b |
0.069 a |
5.76 b |
19.76 a |
0.77 |
75 |
6.70 ab |
0.085 a |
4.24 ab |
15.84 ab |
0.63 |
|
150 |
4.92 a |
0.073 a |
3.50 b |
12.11 b |
0.71 |
|
225 |
5.65 ab |
0.082 a |
3.44 b |
13.18 b |
0.61 |
|
|
|
|
|
|
|
|
H1 |
0 |
3.12 a |
0.080 a |
3.64 a |
11.55 a |
1.17 |
75 |
5.49 a |
0.095 a |
5.57 a |
16.56 a |
1.01 |
|
150 |
4.66 a |
0.074 a |
3.07 a |
12.45 a |
0.66 |
|
225 |
2.96 a |
0.084 a |
2.55 a |
9.60 a |
0.86 |
|
|
|
|
|
|
|
|
H2 |
0 |
8.84 a |
0.086 a |
5.08 a |
19.23 b |
0.57 |
75 |
8.34 ab |
0.080 a |
4.76 a |
19.46 b |
0.57 |
|
150 |
8.76 b |
0.079 a |
5.33 a |
21.38 b |
0.61 |
|
225 |
6.67 a |
0.085 a |
4.18 a |
14.96 a |
0.63 |
|
|
|
|
|
|
|
|
H3 |
0 |
9.95 b |
0.062 a |
7.91 a |
23.77 b |
0.79 |
75 |
8.74 ab |
0.072 a |
6.01 a |
20.99 b |
0.69 |
|
150 |
8.92 ab |
0.064 a |
8.39 a |
25.29 b |
0.94 |
|
225 |
7.72 a |
0.078 a |
5.57 a |
18.99 a |
0.72 |
|
|
|
|
|
|
|
|
H4 |
0 |
6.26 a |
0.072 a |
5.22 b |
15.70 a |
0.83 |
75 |
5.64 a |
0.078 a |
3.32 a |
13.10 a |
0.59 |
|
150 |
6.96 a |
0.081 a |
3.41 a |
15.97 a |
0.49 |
|
225 |
6.28 a |
0.073 a |
3.04 a |
14.32 a |
0.48 |
Means within the column followed by the same letter are not significantly different at P = 5% level, using Duncan’s test
3.2. Tissue mineral content
Salt stress caused a significant increase in accumulation of Na+ and Cl- in both old and current leaves (figure 1 and 2). At 150 and 225 mM, Na+ concentrations increased significantly, compared to control plants, in old leaves more than in current leaves of different hybrids H1, H2, H3 and H4. However, at these same doses, Na+ contents were higher in the current than in the old leaves of ‘Chemlali Sfax’ cultivar (figure 1). Chloride leaf concentration ranged from 0.08 to 0.14 % of dry weight and was dependent on the interaction between cultivar and salinity (figure 2). The higher increased rate (163.7%) of Cl- contents was recorded at 225 mM in the old leaves of hybrid H2. However, the lowest rate (111.8%) was observed in the current leave of hybrid H1.
|
Figure 1: Sodium (Na) concentrations in old (FA) and current (FJ) leaves of five olive cultivars grown at four levels of NaCl (0, 75, 150 and 225 mM) for 180 days.
|
In contrast, leaf potassium (K) concentration decreased as salinity increased (figure 3). Results showed that a linear and a significant decreased (R2 = 0.96) of K contents in old leave were observed for ‘Chemlali Sfax’ cultivar and hybrids H1 and H2. While, for hybrid H4, the increase of K concentration in old and current leaves at 225 mM were respectively 143.3 and 154.5%, comparing to the control plants.
The higher levels of both Na+ and Cl- contents recorded in old than in current leaves confirm the findings of Chartzoulakis (2005) who showed that the greatest decrease in K+ concentration occurs in old leaves and that the olive tree is able to maintain high level of K+ in young leaves. These tendencies seem to be similar with those of the salt-stressed ‘Chemlali’ olive tree. In fact, even stressed, this cultivar was able to maintain its photosynthetic activity, even at low rates (Ben Ahmed et al., 2012).
Also, the salt tolerance of the ‘Chemlali’ cultivar is associated with its ability to exclude the major part of incoming salts at the old leaves, and to avoid Na+ and/or Cl- accumulation in actively growing tissues.
|
Figure 2: Chloride (Cl) concentrations in old (FA) and current (FJ) leaves of five olive cultivars grown at four levels of NaCl (0, 75, 150 and 225 mM) for 180 days. |
|
Figure 3: Potassium (K) concentrations in old (FA) and current (FJ) leaves of five olive cultivars grown at four levels of NaCl (0, 75, 150 and 225 mM) for 180 days. |
3.3. Electrolyte leakage
Cell membrane stability has been widely used to express stress tolerance, and higher membrane stability could be correlated with abiotic stress tolerance (Bajji et al., 2001) and with tested cultivar (Perica et al., 2008). Statistical analysis showed that there were significant differences between all the treatments in the case of new varieties H2, H3 and H4. Indeed, for these cultivars, results showed that salinity increased progressively the electrolyte leakage, respectively, from 22.6 to 60.7%, from 32.6 to 64% and from 27.2 to 61.5% as salinity increased from 0 to 225 mM. However, for ‘Chemlali Sfax’ and H1 cultivars, the electrolyte leakage was unaffected by salinity at 75 and 150 mM. But, under high salinity stress (225 mM), the electrolyte leakage increased significantly with an average of 185% compared to control trees, suggesting membrane permeability damage (figure 4).
|
Figure 4: The effect of the salt stress on electrolyte leakage (% of total electrolyte leakage) of five olive cultivars grown at four levels of NaCl (0, 75, 150 and 225 mM) for 180 days. (Means followed by the same letter are not significantly different at P = 5% level, using Duncan’s test). |
3.4. Proline content
Salt-stress induced caused a significant increase of proline content in leaves of stresses plants (figure 5). The highest accumulations (3.3 and 3.5% µmol/g fresh mass) were observed at 225 mM, respectively, in leaves of hybrids H1 and H3. In moderately salt-stressed plants (150 mM), the proline leaf content of ‘Chemlali Sfax’ cultivar was at more than 2.5 times higher than that recorded in controls but more than 3 times higher under the dose 225 mM. Several papers showed that, in order to accommodate the ionic balance in the vacuoles, the salt stressed olive trees tend to accumulate proline at high levels to improve water uptake to actively growing tissues (Hasegawa et al, 2000). Similarly, the accumulation of proline in the olive tree constitutes other adaptive mechanism to harmful stress.
3.5. Relative water content (RWC)
Water status is highly sensitive to salinity and is dominant in determining the plant responses to stress (Stepien and Klobus, 2006). Generally, a reduction in water content under osmosis stress is the first clear effect on olive trees (Ennajeh et al., 2008; Ben Ahmed et al., 2012). In the present study, the degree of reduction depended on studied cultivar (figure 6). Indeed, six months of salinization were sufficient to decrease the RWC of both cultivars (H2 and H4), and this decrease was more evident in plants in the 225 mM than in plants in the 150 mM NaCl treatment. The higher reduction (12.35%) was observed for hybrid H4 in 225 mM. However, no significant differences in RWC between ‘Chemlali Sfax’, H1 and H3 plants were observed during the salt treatment. RWC ranged between 86.7 and 92.9%. As reported elsewhere (Dichio et al. 2005; Ennajeh et al., 2008), olive is capable of high osmotic adjustment leading to a high water potential gradient between leaves and soil. Such mechanism would to help the plants to preserve appropriate leaf water status.
|
Figure 5: The effect of the salt stress on proline content (in µmol/g MF) of five olive cultivars grown at four levels of NaCl (0, 75, 150 and 225 mM) for 180 days. (Means followed by the same letter are not significantly different at P = 5% level, using Duncan’s test). |
|
Figure 6: The effect of the salt stress on relative water content (RWC, in %) of five olive cultivars grown at four levels of NaCl (0, 75, 150 and 225 mM) for 180 days. (Means followed by the same letter are not significantly different at P = 5% level, using Duncan’s test).
|
4. Conclusion
The conducted study confirmed the genetic variability in salt tolerance among tested cultivars. The new olive variety (hybrid H1) issued from a Tunisian breeding crosses was demonstrated to be the most tolerant, showing a lower reduction of growth and the ability to limit leaf Na+ concentration at 150 mM compared to “Chemlali Sfax” variety. However, the hybrids H2 and H3 are considered the most sensitive to the salinity.
Acknowledgments
This research was financially supported by the Tunisian Ministry of Higher Education and Scientific Research (Laboratoire d’Amélioration et de Protection des Resources Génétiques de l’Olivier, Institut de l’Olivier). We wish to thank Yengui A. and Soua N. for their great technical assistance.
5. References
Antipolis S (2003) Les menaces sur les sols dans les pays méditerranéens. Plan Bleu, ISBN: 2-912081-13-0.
Ashraf M, Foolad M.R (2007) Role of glycine betaine and proline in improving plant abiotic stress resistance. Environ. Exp. Bot 59:206-216.
Ashraf M, O’leary J.W (1996) Responses of some newly evolved salt tolerant genotypes of spring wheat to salt stress I: Yield components and ion distribution. J. Agron. Crop. Sci 176:91-101.
Bader B, Aissaoui F, Kmicha F, Ben Salem A, Chehab H, Gargouri K, Boujnah D, Chaieb M (2015) Effects of salinity stress on water desalination, olive tree (Olea europaea L. cvs Picholine, Meski and Ascolana) growth and ion accumulation. Desalination 364:46-52.
Bajji M, Kinet JM, Lutts S (2001) The use of the electrolyte leakage method for assessing cell membrane stability as a water stress tolerance test in durum wheat. Plant Growth Regul 00:1-10.
Bates L.S., Waldem R.P., Teare I.D (1973) Rapid determination of free proline for water stress studies. Plant and Soil 39:205-208.
Ben Ahmed C, Magdich S, Ben Rouina B, Boukhris M, Ben Abdullah F (2012) Saline water irrigation effects on soil salinity distribution and some physiological responses of field grown Chemlali olive. J. Environ. Manage 113:538-544.
Ben Rouina B, Trigui A, Boukhris M (2002) Effect of climate and the soil conditions on crops performance of the Chemlali de Sfax “olive tree”. Acta Hortic 586:285–289.
Chartzoulakhis K.S (2005) Salinity and olive: growth, salt tolerance, photosynthesis and yield. Agric. Water Manage 78:108-121.
Chartzoulakis K, Loupassaki M, Bertaki M, Androulakis I (2002) Effects of NaCl salinity on growth, ion content and CO2 assimilation rate of six olive cultivars. Sci. Hortic 96:235–247.
Dichio B, Xiloyannis C, Sofo A, Montanaro G (2005) Osmotic regulation in leaves and roots of olive trees during a water deficit and rewatering. Tree Physiol 26:179–185.
Ennajeh M, Tounekti T, Vadel A.M., Khemira H, Cochard H (2008) Water relations and drought-induced mebolism in olive (Olea europaea) varieties ‘Meski’ and ‘Chemlali’ during severe drought. Tree Physiol 28 :971-976.
Grati N, Khlif M et al (2001) Caractérisation technologique des variétés d’olivier cultivées en Tunisie. Ezzaitouna. Numéro spécial (ISSN : 0330-6828). 68p.
Gucci R, Lombardini L, Tattini M (1997) Analysis of leaf water relations in leaves of two olive (Olea europaea) cultivars differing in tolerance to salinity. Tree Physiol 17:13-21.
Hasegawa P.M., Bressan R.A, Zhu J.K, Bohnert H.J (2000) Plant cellular and molecular responses to high salinity. Annu. Rev. Plant Physiol. Plan Mol. Biol 51:463-499.
Kchaou H, Larbi A, Gargouri K, Chaieb M, Morales F, Msallem M (2010) Assessment of tolerance to NaCl salinity of five olive cultivars, based on growth characteristics and Na+ and Cl- exclusion mechanisms. Sci. Horti 124:306-315.
Khlif M, Ayadi M, Grati‐Kammoun N, Arous M. N et al (2002) Identifiying “Chemlali” olive variety in its traditional area. Acta Hortic 586:117–120.
Lutts S, Kinet J.M, Bouharmont J (1996) NaCl-induced senescence in leaves of rice (Oryza sativa L.) cultivars differing in salinity resistance. Ann. Bot 78:389-398.
Rugini E, Fedeli E (1990) Olive (Olea europaea L.) as an oilseed crop. In: Bajaj, Y.P.S (ed) Bio-technology in Agriculture and forestry Legume and oilseed crops I, vol 1, Springer, Berlin, pp 593-641.
Perica S, Goreta S, Selak G.V (2008) Growth, biomasse allocation and leaf ion concentration of seven olive (Olea europaea L.) cultivars under increased salinity. Sci. Horti 117:123-129.
Shipley B, Meziane D (2002) The balanced-growth hypothesis and the allometry of leaf and root biomass allocation. Funct. Ecol 16:326-331.
Stepien P, Klobus G (2006) Water relations and photosynthesis in Cucumis sativus L. leaves under salt stress. Biol Plant 50: 610–616.
Tattini M, Bertoni P, Caselli S (1992) Genotypic responses of olive plants to sodium chloride. J. Plant Nutr 15:1467–1485.
Trigui A, Msallem M et al (2001) L’amélioration génétique par croisement dirigé de l’olivier (Olea europaea L.) cv. Chemlali Sfax et Meski. Symposium de clôture du projet AGO (COI/FCPB), Le caire (Egypte) 25-28/06/2001.
Trigui A, Msallem M et al (2002) Oliviers de Tunisie: catalogue des variétés autochtones et types locaux. Vol 1. IRESA, Institut de l’Olivier.