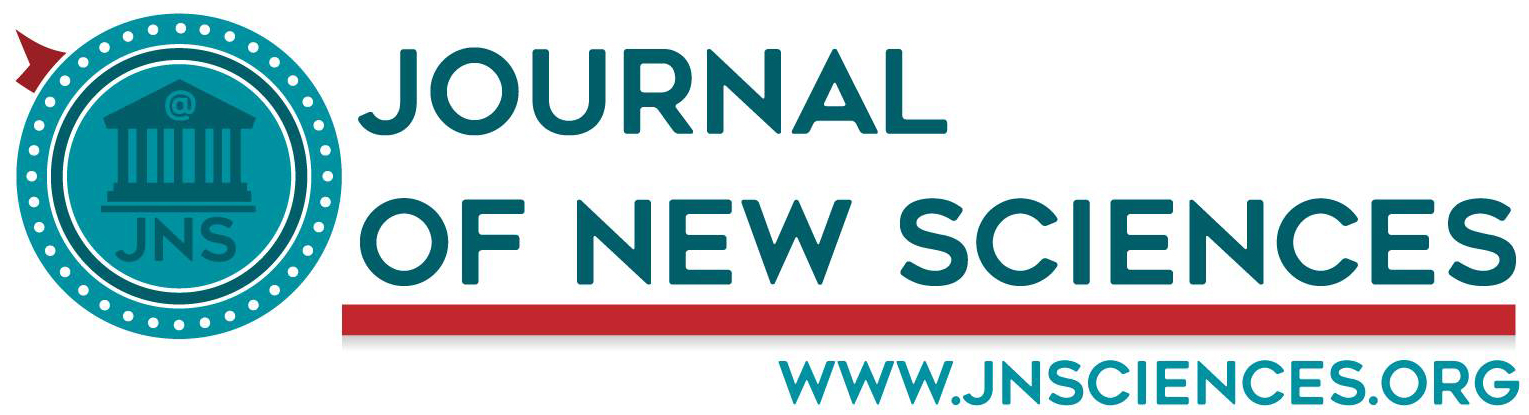
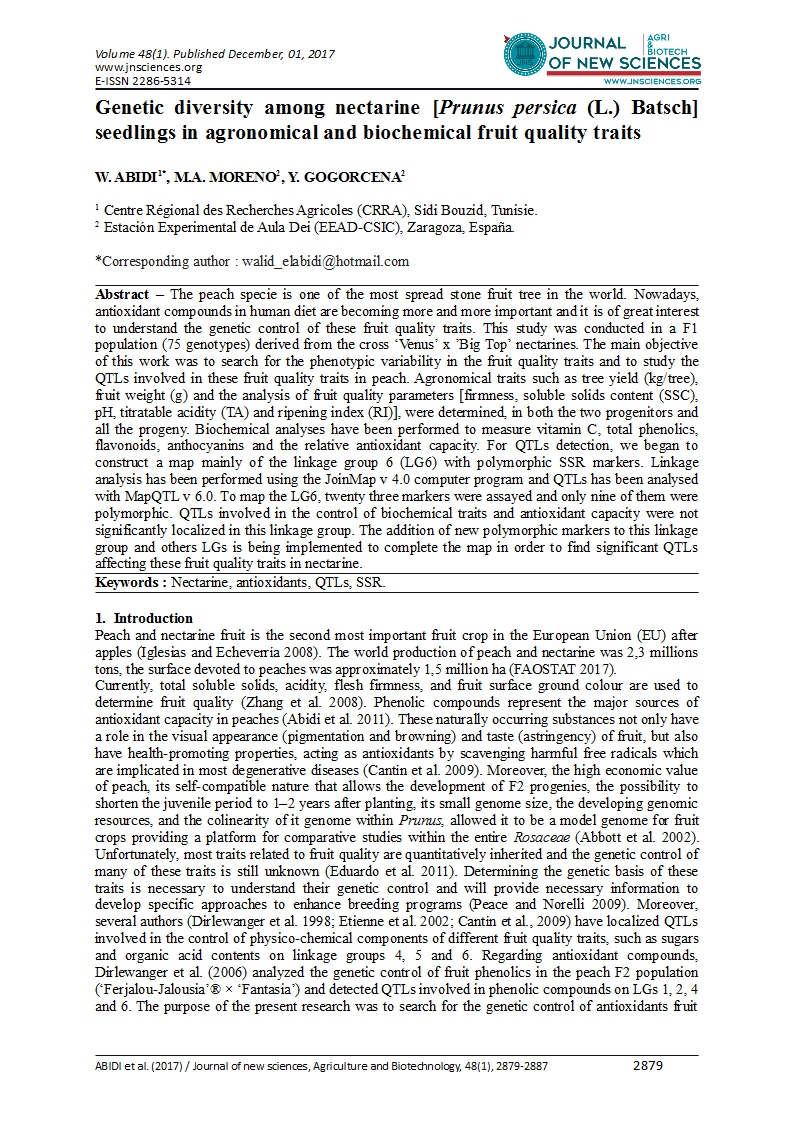
- Category: Volume 48
- Hits: 3838
Genetic diversity among nectarine [Prunus persica (L.) Batsch] seedlings in agronomical and biochemical fruit quality traits
W. ABIDI1*
M.A. MORENO2
Y. GOGORCENA2
1 Centre Régional des Recherches Agricoles (CRRA), Sidi Bouzid, Tunisie.
2 Estación Experimental de Aula Dei (EEAD-CSIC), Zaragoza, España.
Abstract – The peach specie is one of the most spread stone fruit tree in the world. Nowadays, antioxidant compounds in human diet are becoming more and more important and it is of great interest to understand the genetic control of these fruit quality traits. This study was conducted in a F1 population (75 genotypes) derived from the cross ‘Venus’ x ’Big Top’ nectarines. The main objective of this work was to search for the phenotypic variability in the fruit quality traits and to study the QTLs involved in these fruit quality traits in peach. Agronomical traits such as tree yield (kg/tree), fruit weight (g) and the analysis of fruit quality parameters [firmness, soluble solids content (SSC), pH, titratable acidity (TA) and ripening index (RI)], were determined, in both the two progenitors and all the progeny. Biochemical analyses have been performed to measure vitamin C, total phenolics, flavonoids, anthocyanins and the relative antioxidant capacity. For QTLs detection, we began to construct a map mainly of the linkage group 6 (LG6) with polymorphic SSR markers. Linkage analysis has been performed using the JoinMap v 4.0 computer program and QTLs has been analysed with MapQTL v 6.0. To map the LG6, twenty three markers were assayed and only nine of them were polymorphic. QTLs involved in the control of biochemical traits and antioxidant capacity were not significantly localized in this linkage group. The addition of new polymorphic markers to this linkage group and others LGs is being implemented to complete the map in order to find significant QTLs affecting these fruit quality traits in nectarine.
Keywords : Nectarine, antioxidants, QTLs, SSR.
1. Introduction
Peach and nectarine fruit is the second most important fruit crop in the European Union (EU) after apples (Iglesias and Echeverría 2008). The world production of peach and nectarine was 2,3 millions tons, the surface devoted to peaches was approximately 1,5 million ha (FAOSTAT 2017).
Currently, total soluble solids, acidity, flesh firmness, and fruit surface ground colour are used to determine fruit quality (Zhang et al. 2008). Phenolic compounds represent the major sources of antioxidant capacity in peaches (Abidi et al. 2011). These naturally occurring substances not only have a role in the visual appearance (pigmentation and browning) and taste (astringency) of fruit, but also have health-promoting properties, acting as antioxidants by scavenging harmful free radicals which are implicated in most degenerative diseases (Cantín et al. 2009). Moreover, the high economic value of peach, its self-compatible nature that allows the development of F2 progenies, the possibility to shorten the juvenile period to 1–2 years after planting, its small genome size, the developing genomic resources, and the colinearity of it genome within Prunus, allowed it to be a model genome for fruit crops providing a platform for comparative studies within the entire Rosaceae (Abbott et al. 2002). Unfortunately, most traits related to fruit quality are quantitatively inherited and the genetic control of many of these traits is still unknown (Eduardo et al. 2011). Determining the genetic basis of these traits is necessary to understand their genetic control and will provide necessary information to develop specific approaches to enhance breeding programs (Peace and Norelli 2009). Moreover, several authors (Dirlewanger et al. 1998; Etienne et al. 2002; Cantín et al., 2009) have localized QTLs involved in the control of physico-chemical components of different fruit quality traits, such as sugars and organic acid contents on linkage groups 4, 5 and 6. Regarding antioxidant compounds, Dirlewanger et al. (2006) analyzed the genetic control of fruit phenolics in the peach F2 population (‘Ferjalou-Jalousia’® × ‘Fantasia’) and detected QTLs involved in phenolic compounds on LGs 1, 2, 4 and 6. The purpose of the present research was to search for the genetic control of antioxidants fruit quality traits in a population of nectarines that include 75 different genotypes, derived from the cross ‘Venus’ x ’Big Top’.
2. Material and Methods
2.1. Plant material
The population assayed was a segregant F1 population (75 individual tree seedlings) obtained from a controlled intra-specific cross made in 2000-2001 between Prunus persica cvs. ‘Venus’ (female parent) and ‘BigTop’ (male parent), in collaboration with Agromillora Catalana S.A. ‘Venus’ is a freestone melting flesh (FMF) nectarine cultivar whereas ‘Big Top’ is a clingstone melting flesh (CMF) nectarine cultivar. The segregant population is entirely melting flesh, either cling- or freestone. Seedlings were established during 2001 in a plot at the Experimental Station of Aula Dei (Zaragoza, Spain).
2.2. Agronomic fruit quality traits
Fruits were handpicked at commercial maturity, assessed by peel fruit colour and firmness. Total yield (kg/tree) was determined for each individual seedling tree recording also the total number of fruits. For the evaluation of fruit quality parameters, a representative sample of twenty fruits per tree was selected. Flesh firmness was determined with a penetrometer fitted with an 8-mm diameter probe. The soluble solids content (SSC) of the juice was measured with a temperature compensated refractometer (model ATC-1, Atago Co., Tokyo, Japan); and data are given as ºBrix. The titratable acidity (TA) was measured by titration of 5 ml of juice with NaOH 0.1N to pH 8.1 (AOAC 1984). Ripening index (RI) was calculated as the ratio of SSC to TA. Then, 5 g flesh samples were prepared for each seedling tree, frozen in liquid nitrogen and stored at -20ºC until analyses.
2.3. Biochemical analyses
The frozen fruit material (5 g) was incubated overnight at 4 ºC and homogenized with a Polytron (2 min on ice) with 10 ml of extraction solution, consisting of 0.5 N HCl in methanol / Milli-Q water (80% v/v). The mixture was then centrifuged for 20 min at 4 ºC and 16,000 rpm. Supernatant was recovered and the volume measured. This hydroalcoholic extract was used for total phenolics, anthocyanins, flavonoids, and antioxidant capacity assays. For the determination of ascorbic acid, samples were kept overnight at 4ºC in 10 ml 5 % metaphosphoric acid, then homogenized and centrifuged as it was described above.
2.3.1. Vitamin C
To measure vitamin C, the procedures used were as described by Law et al. (1983), adapted from Okamura et al. (1980) for the spectrophotometric determination of ascorbic acid. Absorbance was measured at 525 nm using a spectrophotometer (Beckman Coulter DU 800). The standard calibration curve was daily prepared using ascorbic acid as standard. Vitamin C was expressed as mg ascorbic acid (AA) per 100 g FW.
2.3.2. Total phenolics
The total phenol content in fruits was evaluated according to Swain and Hills method (1959), the assay consists of a colorimetric method based on the chemical reduction of the Folin-Ciocalteau reagent. Absorbance was measured at 725 nm using a spectrophotometer (Beckman Coulter DU 800). The phenolic content was expressed in milligrams of gallic acid equivalents (GAE) per 100 g fresh weight (FW).
2.3.3. Flavonoids
Total flavonoids content was determined using a colorimetric assay based on the method of Zhishen et al. (1999). Absorbance was measured at 510 nm against a blank with a spectrophotometer (Beckman Coulter DU 800). The results were expressed as milligrams of catechin equivalents (CE) per 100 g of FW based on a standard curve using catechin as standard.
2.3.4. Anthocyanins
Total anthocyanin content was evaluated using the method of Fuleki and Francis (1968) adapted to peach tissue. Absorbance of the extract was measured at 535 and 700 nm. The anthocyanins concentration in the original sample was calculated in mg cyaniding 3-glucoside equivalent /kg of FW using the molar extinction absorptivity coefficient = 25,965 cm-1 M-1.
2.3.5. Relative antioxidant capacity (RAC)
Antioxidant capacity of peach extracts was measured using the 1, 1-diphenyl-2-picrylhydrazyl (DPPH), as described by Brand-Williams et al. (1995). The absorbance of samples was measured at 515 nm after 10 min of reaction. The antioxidant activity was expressed as µg Trolox/g of FW.
2.4. DNA extraction and molecular analysis
Young fresh leaves were collected from each individual seedling tree, frozen and ground with liquid nitrogen, and samples were stored at -20ºC until extraction. DNA was extracted from leaves of ‘Venus’, ‘Big Top’ and all the progeny (75 genotypes) by using the DNeasy Plant Mini Kit (QIAGEN Inc., Valencia, CA), following manufactured instructions. For initial polymorphism testing, forty markers were used (Table 1.) and assays were performed on ‘Venus’, ‘Big Top’, and six progenies. For the PCR reactions, 10 ng of genomic DNA was amplified in a final volume of 15 µl containing 1X biotools buffer, 2.0 mM MgCl2, 0.20 mM of dNTPs, 0.15 µM of each primer pair and 0.5 U Taq DNA polymerase (Biotools, Madrid, Spain). The PCR conditions were as follows: preliminary denaturation (3 min at 94 ºC), followed by 35 cycles consisting of denaturation (1 min at 94 ºC), 45 s at the corresponding annealing temperature and extension (1 min at 72 ºC), and a final extension after the last cycle (7 min at 72 ºC) (Gene Amp 2700 thermocycler). After amplification, acrylamide gels were prepared and 0.5 µl of the PCR product for each genotype was loaded either in acrylamide or on “LI-Cor automatic sequencer” and data were collected. Fragment sizes were estimated with the 100-bp ladder-DNA-Brand sizing markers. Subsequent analyses were performed on all progeny, including selfs (individuals: 1, 11, 15, 25, 57 and 72), using the nine primers that were polymorphic for the LG 6.
Table 1. Characteristics of the SSR markers tested for polymorphism in the progeny
|
|||||
Locus code |
Reference |
LGa |
PMb |
AT (ºC) |
bp |
BPPCT002 |
Dirlewanger et al., 2002 |
2 |
M |
57 |
229 |
BPPCT007 |
Dirlewanger et al., 2002 |
3 |
P |
57 |
149 |
BPPCT008 |
Dirlewanger et al., 2002 |
6 |
M |
57 |
140-160 |
BPPCT017 |
Dirlewanger et al., 2002 |
5 |
M |
57 |
174 |
BPPCT018 |
Dirlewanger et al., 2002 |
6 |
P |
57 |
222 |
BPPCT019 |
Dirlewanger et al., 2002 |
8 |
M |
57 |
194 |
BPPCT020 |
Dirlewanger et al., 2002 |
1 |
M |
57 |
200 |
BPPCT021 |
Dirlewanger et al., 2002 |
3 |
M |
57 |
240 |
BPPCT024 |
Dirlewanger et al., 2002 |
2 |
M |
57 |
96 |
BPPCT025 |
Dirlewanger et al., 2002 |
6 |
P |
57 |
155-175 |
BPPCT033 |
Dirlewanger et al., 2002 |
6 y 8 |
P |
57 |
180 |
CPPCT030 |
Aranzana et al., 2002 |
6 |
P |
52 |
170-200 |
CH15 |
Struss et al., 2003 |
- |
P |
52 |
85-95 |
Chill-0593 |
KAC, unpublished |
6 |
P |
- |
- |
Chill-PPN12C07 |
KAC, unpublished |
6 |
P |
- |
- |
Chill-0328 |
KAC, unpublished |
6 |
M |
- |
- |
Chill-0402 |
KAC, unpublished |
6 |
M |
- |
- |
Chill-1182 |
KAC, unpublished |
6 |
M |
- |
- |
Chill-1294 |
KAC, unpublished |
6 |
M |
- |
- |
Chill-PPN07E01 |
KAC, unpublished |
6 |
M |
- |
- |
Chill-PPN31E06 |
KAC, unpublished |
6 |
M |
- |
- |
EPPCU6377 |
Howard et al., 2005 |
8 |
P |
55 |
123 |
PAL |
KAC, unpublished |
6 |
M |
- |
- |
PGIP |
KAC, unpublished |
6 |
M |
- |
- |
pchcms5 |
Abbott et al., 2000 |
6 |
P |
52 |
246 |
SOD-4A |
KAC, unpublished |
6 |
M |
- |
- |
UDP96-001 |
Testolin et al., 1999 |
6 |
M |
57 |
120 |
UDP96-003 |
Testolin et al., 1999 |
4 |
M |
57 |
85-115 |
UDP96-005 |
Testolin et al., 1999 |
1 |
M |
57 |
82-164 |
UDP96-010 |
Testolin et al., 1999 |
6 |
M |
57 |
131 |
UDP96-018 |
Testolin et al., 1999 |
1 |
M |
57 |
242-282 |
UDP96-019 |
Testolin et al., 1999 |
8 |
M |
57 |
170-214 |
UDP97-401 |
Testolin et al., 1999 |
5 |
M |
57 |
130 |
UDP98-021 |
Testolin et al., 1999 |
6 |
M |
57 |
- |
UDP98-022 |
Testolin et al., 1999 |
1 |
M |
57 |
- |
UDP98-024 |
Testolin et al., 1999 |
4 |
P |
57 |
105 |
UDP98-025 |
Testolin et al., 1999 |
2 |
M |
65 |
101-159 |
UDP98-407 |
Testolin et al., 1999 |
6 |
P |
60 |
180-190 |
UDP98-410 |
Testolin et al., 2000 |
5 |
M |
57 |
135-185 |
UDP98-412 |
Testolin et al., 1999 |
6 |
P |
57 |
95-140 |
UDP98-416 |
Testolin et al., 1999 |
6 |
M |
57 |
80-90 |
2.5. Mapping, QTL and statistical analysis
Nine polymorphic microsatellite markers were used to construct a partial map of the LG 6 with JoinMap 4.0 software (Van Ooijen 2006). QTL analysis was implemented with MapQTL 6.0 (Van Ooijen 2009) by interval mapping. Statistical analyses were performed using SPSS 20.0 to obtain basic statistics for the entire plant material studied. Maximum and minimum values, mean, mean standard deviation (MSE) and correlations between all traits were calculated.
3. Results and Discussion
3.1. Agronomic and fruit quality traits
Several agronomic and fruit quality traits were evaluated. Mean values of production, fruit firmness, soluble solids content (SSC), titratable acidity (TA) and the ripening index ratio (RI = SSC/TA) for the progeny and the two progenitors are described in Table 2.
Table 2. Agronomic and basic fruit quality traits of the ‘Venus’ x ‘Big Top’ population
|
|||||
|
Progenitors |
Progeny |
|||
Traits |
‘Venus’ |
‘Big Top’ |
Min |
Max |
Mean ± SD |
Fruit weight (g) |
333 |
156 |
70 |
250 |
173 ± 35 |
Firmness (N) |
37 |
40 |
27.4 |
60.7 |
44 ± 6.5 |
SSC (ºBrix) |
14.0 |
14.5 |
9.2 |
18.2 |
13.5 ± 1.9 |
pH |
3.5 |
3.9 |
3.4 |
4.4 |
3.80 ± 0.3 |
TA |
0.9 |
0.5 |
0.3 |
0.9 |
0.6 ± 0.2 |
RI (SSC/TA) |
14.9 |
26.4 |
11.5 |
46.9 |
26 ± 10 |
SSC= soluble solids content (ºBrix), TA= titratable acidity, RI = ripening index (SSC/TA) |
Mean values was calculated from 75 individual seedlings values. The progeny produced low yield with high variability among genotypes ranging from 1.2 to 19.5 kg per tree with a mean of 8.4 kg. Also, the fruit number per tree showed high variability and ranged from 6 to 100 fruits. As a consequence of the variability in tree production and fruit number, the fruit weight varied greatly among genotypes, and ranged from 70 to 250 g with a mean value of 173 ± 35 g.
Flesh firmness ranged from 27 to 60 N with a mean value of 44 ± 6.5 N. The mean firmness obtained was 44 N (4.5 kg) which is lower than the maximum levels of fruit firmness for marketing fresh peaches and nectarines, set by the EU at a 6.5 kg/0.5 cm2 (= 63.7 N), using a 8 mm diameter probe (Commission Regulation EC, No.1861/2004 of 28 October 2004). Di Vaio et al. (2008) reported that mean flesh firmness was 46.9 N for nectarines and 42.9 N for peaches in 13 peach and nectarine cultivars.
The SSC values obtained ranged from 9.2 to 18.2 ºBrix, but in all cases were greater than 8 ºBrix, which is the minimum established by the EU to market peaches and nectarines (R-CE No.1861/2004). Di Vaio et al. (2008) reported that SSC in nectarine cultivars was on average higher (11.4 °Brix) than in peaches (10.2 °Brix) in 13 peach and nectarine cultivars.
Regarding acidity (TA), values ranged from 0.3 to 0.9 with a high TA mean among genotypes (0.6 ± 0.2). The parental `Venus´ which is an acid fruit showed the highest values of TA. For the ripening index, values ranged from 11.5 to 46.9 depending on their SSC and TA. A high variability was also observed among genotypes (26 ± 10) in the progeny. The TA plays an important role at low SSC levels (<10%). Crisosto et al. (1997) reported that peaches consumer acceptance is closely related to acid content as well as fruit firmness. However, stone fruits lose acidity during the maturation and ripening process. This maturity feature is also affected by cultivar and seasonal variability (Crisosto 1994).
3.2. Biochemical analyses
Table 3 shows the values for all the antioxidant compounds determined as well as the antioxidant capacity, in the ‘Venus’ x ‘Big Top’ progeny.
Table 3. Variation in the content of antioxidant compounds in the studied population |
|||||
|
Progenitors |
Progeny |
|||
Compounds |
‘Venus’ |
‘Big Top’ |
Min |
Max |
Means ± SD |
Vitamin C (mg AA /100 g of FW) |
3.3 |
5.6 |
1.3 |
9.5 |
3.9 ± 1.7 |
Total phenolics (mg GAE/100 g of FW) |
36.5 |
46.6 |
23.0 |
51.2 |
44.0 ± 6.1 |
Flavonoids (mg CE/100g of FW) |
13.7 |
16.9 |
5.0 |
47.0 |
21.2 ± 9.4 |
Anthocyanins (mg C3G eq /kg of FW) |
1.9 |
1.4 |
0.3 |
5.0 |
1.7 ± 0.7 |
Antioxidant capacity (μg Trolox/g of FW) |
423.0 |
926.2 |
255.0 |
1099.5 |
617.0 ± 200 |
AA= Ascorbic acid; C3G eq = Cyanidin-3-glucoside equivalents; CE= catechin equivalents; GAE= gallic acid equivalents. |
The results obtained for all biochemical traits showed a normal distribution typical of quantitative characters (Figure 1).
3.2.1. Vitamin C
The ascorbic acid (Vitamin C) content ranged in the progeny from 1.3 to 9.5 mg of AA/100g of FW, with a mean value of 3.9 mg of AA/100 g of FW (Table 3). The columns in the Figure 3.1 represent the segregation values of ascorbic acid and the number of genotypes for each range. The parents segregated for Vitamin C content, and as a consequence the progeny showed high variation among genotypes. Cantín et al.(2009a) reported that the total ascorbic acid (vitamin C) content varied greatly from approximately 1 to 9 mg of AA/100 g of FW, with a mean value of 3.7 mg of AA/100 g of FW in 218 peach genotypes.
3.2.2. Total phenolics content
Total phenolics ranged from 23 to 51 mg GAE/100g of FW (Table 3.2) with a mean value of 44.0 mg GAE/100g of FW. Columns in Figure 1 represent the distribution of total phenolics in the progeny and the number of genotypes for every range. Cantín et al.(2009a) also reported that total phenolics varied among cultivars and genotypes with obtained values in the range of 12.7-71.3 mg of GAE/100 g of FW.
3.2.3. Flavonoids content
Figure 1 showed the normal distribution of flavonoids in the progeny. This trait ranged from 5 to 47 mg CE/100g of FW (Table 3) with an average of 21 mg of CE/100 g of FW. Marinova et al. (2005) reported that flavonoids content for peach was 15.0 mg CE/100 g of FW, which is very close to our progeny (21.2 mg CE/100 g of FW). Cantín et al. (2009) reported that total flavonoids content in 218 peaches ranged from 1.8 to 30.9 mg of CE/100 g of FW.
3.2.4. Anthocyanins content
Anthocyanins content also showed a normal distribution and ranged from 0.3 to 5 mg C3G eq/kg of FW (Table 3) with an average of 1.7 mg C3G eq/kg. Cantin et al.(2009) reported that total anthocyanins varied greatly among genotypes from 0.1 to 26.7 mg of cyanidin-3-glucoside equivalents (C3G) per kg of FW, depending on the red pigmentation of the flesh.
3.2.5. Relative antioxidant capacity (RAC)
Figure 1 represents the distribution of the relative antioxidant capacity (RAC) in the progeny and the number of genotypes in each range. The antioxidant capacity ranged from 255 to 1099 μg Trolox/g of FW (Table 3) with an average of 617.0 μg Trolox/g of FW. The parents, ‘Venus’ and ‘Big Top’, showed a clear segregation for RAC and the progeny showed a high variation.
The relative antioxidant capacity (RAC) in our progeny varied greatly among genotypes, reaching a maximum value of 1100 μg of Trolox/g of FW. Cantín et al. (2009a) reported that the RAC varied among 218 genotypes, with values ranging from 227.3 to 629.9 μg of Trolox/g of FW, with an average of 405 μg of Trolox/g of FW.
|
Figure 1. Segregation of antioxidant compounds in the `Venus´ x `Big Top´ progeny. Arrows show the range for the parents ‘Venus’ (‘V’) and ‘Big Top’ (‘BT’).
|
3.3. Correlations between traits
Table 4 shows the correlation coefficients between biochemical traits. Total phenolics presented significant positive correlations at P ≤ 0.01 with flavonoids (r = 0.619), ascorbic acid (r = 0.426), and RAC (r = 0.485), implying that both flavonoids and ascorbic acid are implicated in the antioxidant activity of peaches. Cantín et al. (2009a) reported, also in peach genotypes, that flavonoids had a high positive correlation with total phenolics (r = 0.742**) and the relative antioxidant capacity (r = 0.553**). Also, the ascorbic acid showed positive significant correlations with flavonoids (r = 0.374**), total phenolics (r = 0.426**) and relative antioxidant capacity (r = 0.316**). Results obtained in some genotypes of the same population and others did not show correlation of vitamin C versus any other phytochemical trait (Cantín et al. 2009a).
Table 4. Pearson´s correlation coefficients between phytochemical traits
|
|||
Traits |
Vitamin C |
Flavonoids |
RAC |
Vitamin C |
|
0.374** |
0.316** |
Total phenolics |
0.426** |
0.619** |
0.485** |
Flavonoids |
|
|
0.814** |
**, P ≤ 0.01; ns, not significant. Abbreviations: RAC, Relative Antioxidant Capacity |
3.4. Mapping and QTL analysis
For QTLs detection, the partial map of the LG 6 was constructed (Figure 2). Only six polymorphic SSR markers were mapped, of the twentythree SSR markers, for LG6 studied. Another three polymorphic markers (BPPCT018, BPPCT033 and UDP98-412) scored in the population, were not mapped in this LG 6 as a consequence of the high distance which they have with the rest of markers, and so they cannot be joined in this map. The addition of new polymorphic markers is being implemented, as well as the construction of new LG in order to find other regions responsible for the genetic control of these biochemical traits. Our preliminary results have not allowed identifying any QTLs involved in these traits in the LG6. We continue mapping other linkage groups in order to locate QTLs controlling these biochemical traits.
|
Figure 2. Linkage map of peach chromosome 6 (LG 6) in the ‘Venus’ x ‘Big Top’ progeny (on the left side, distance in cM, on the right side: markers see Table 2.1 for description).
|
Different authors have shown QTLs involved in agronomic traits such as blooming, ripening and fruit quality traits such as fresh weight, colour, pH, titratable acidity, SSC, sucrose, sorbitol, malic and citric acids, some of them previously identified in the LG6 (Dirlewanger et al. 1999 and Etienne et al. 2002). In our progeny, only 6 polymorphic SSR were included in the partial map of Linkage group 6. Until now, significant QTLs for fruit quality traits were not observed in this LG6. Nevertheless, we are studying other markers in this LG6 and others LGs in order to find significant QTLs related to fruit quality traits.
4. Conclusion
The progeny showed a great phenotypic variance for all the studied traits, such as yield, fruit weight, firmness, soluble solids content, titratable acidity, total phenolics, flavonoids, anthocyanins, ascorbic acid and antioxidant capacity. The significant correlations found between some agronomic and quality attributes could be of interest for quality oriented fruit breeding programs. Six polymorphic SSR markers from a total of twentythree were mapped in the LG6. The addition of new polymorphic markers to this linkage group and others LGs is being implemented to complete the map and to find significant QTLs involved in the antioxidant capacity and fruit quality traits in peaches.
Acknowledgments
The authors thank T. Buhner, C. Cantín, R. Giménez and E. Sierra for technical assistance and support. This study was supported by the Spanish MICINN (Ministry of Science and Innovation) grants AGL-2008-00283. W. Abidi was supported by a JAE-Pre-fellowships from CSIC, Spain.
5. References
Abbott AG, Georgi L, Inigo M, Sosinski B, Yvergniaux D, Wang Y, Blenda A, Reighard G (2002) Peach: the model genome for Rosaceae. Sci. Hort., 575: 145 –155.
Abidi W, Jiménez S, Moreno MÁ, Gogorcena Y (2011) Evaluation of antioxidant compounds and total sugar content in a nectarine [Prunus persica (L.) Batsch] progeny. Inter. J. Mol. Sci 12 (10): 6919-6935
AOAC (1984) Official Methods of Analysis oftheAssociation of the OfficialAnalytical Chemists (Ed.S. Williams). Assoc. Biol. Anal. Chern. Inc. Ltd.: 1141
Brand-Williams W, Cuvelier ME, Berset C (1995) Use of free radical method to evaluate antioxidant activity. Food Sci. Technol., (London), 28: 25–30.
Cantín C, Moreno MA, Gogorcena Y (2009a) Evaluation of the antioxidant capacity, phenolic compounds, and vitamin C content of different peach and nectarine [Prunus persica (L.) Batsch] breeding progenies. J. Agric. Food Chem., 57: 4586–4592.
Crisosto CH (1994) Stone fruit maturity indices: a descriptive review. Postharvest. News Inf., 5, 6: 65-68.
Crisosto CH, Johnson RS, DeJong T, Day KR (1997) Orchard factors affecting postharvest stone fruit quality. Hort Sci., 32: 820–823.
Di Vaio C, Graziani G, Marra L, Cascone A, Ritieni A (2008) Antioxidant capacities, carotenoids and polyphenols evaluation of fresh and refrigerated peach and nectarine cultivars from Italy. Eur. Food Res. Technol., 227: 1225–1231.
Dirlewanger E, Cosson P, Boudehri K, Renaud C, Capdeville G, Tauzin Y, Laigret F, Moing A (2006) Development of a second generation genetic linkage map for peach [Prunus persica (L.) Batsch] and characterization of morphological traits affecting flower and fruit. Tree Genet. Geno., 3: 1–13.
Dirlewanger E, Moing A, Rothan C, Svanella L, Pronier V, Guye A, Plomion C, Monet R (1999) Mapping QTL controlling fruit quality in peach [Prunus persica (L.) Batsch]. Theor. Appl. Genet., 98: 18–31.
Dirlewanger E, Pronier V, Parvery C, Rothan C, Guy A, Monet R (1998) Genetic linkage map of peach [Prunus persica (L.) Batsch] using morphological and molecular markers. Theor. Appl. Genet. 97, 888-895.
Eduardo I, Pacheco I, Chietera G, Bassi D, Pozzi C, Vecchietti A, Rossini L (2011) QTL analysis of fruit quality traits in two peach intraspecific populations and importance of maturity date pleiotropic effect. Tree Genet Genomes 7:323–335
Etienne C, Dirlewanger E, Cosson P, Svanella-Dumas L, Monet R, Moing A, Rothan C, Bodénès C, Plomion C, Kervella J (2002) QTLs and genes controlling peach fruit quality. Acta Hort., 592: 253–258.
FAOSTAT (2017) http://faostat.fao.org
Fuleki T, Francis FJ (1968) Quantitative methods for anthocyanins. 2. Determination of total anthocyanin and degradation index for cranberry juice. J. Food Sci., 33: 78–83.
Iglesias I, Echeverría G (2008) Differential effect of cultivar and harvest date on nectarine colour, quality and consumer acceptance I. Acta Hort., 120: 41–50.
Law MY, Charles SA, Halliwell B (1983) Glutathione and ascorbic acid in spinach (Spinacea oleracea) chloroplasts. The effect of hydrogen peroxide and of paraquat. Biochemistry Journal 210: 899–903.
Marinova D, Ribarova F, Atanassova M (2005) Total phenolics and total flavonoids in Bulgarian fruits and vegetables. Journal of the University of Chemical Technology and Metallurgy, 40, 3: 255–260.
Okamura M (1980) An improved method for determination of L-ascorbic acid and L-dehydroascorbic acid in blood plasma. Clin. Chim. Acta., 103: 259.
Peace C, Norelli J (2009) Genomics approaches to crop improvement in the Rosaceae. In: Folta KM, Gardiner SE (eds) Genetics and genomics of Rosaceae, vol 6. Plant genetics and genomics: crops and models. Springer, New York, pp 19–53
Swain T, Hillis W (1959) The phenolic constituents of Prunus domestica. I. The quantitative analysis of phenolic constituents. J. Sci. Food Agric., 10: 63–68.
Van Ooijen JW (2006) JoinMap 4, Software for the calculation of genetic linkage maps in experimental populations. Kyazma B.V., Wageningen, Netherlands.
Van Ooijen JW (2009) MapQTL 6, Software for the mapping of quantitative trait loci in experimental populations of diploids species. Kyazma B.V., Wageningen, Netherlands.
Zhang Q, Yan G, Dai H, Zhang X, Li C, Zhang Z (2008) Characterization of Tomentosa cherry (Prunus tomentosa Thunb.) genotypes using SSR markers and morphological traits. Sci. Hort. 118: 39–47.
Zhishen J, Mengcheng T, Jianming W (1999) The determination of flavonoid contents in mulberry and their scavenging effects on superoxide radicals. Food Chem., 64: 555–559.