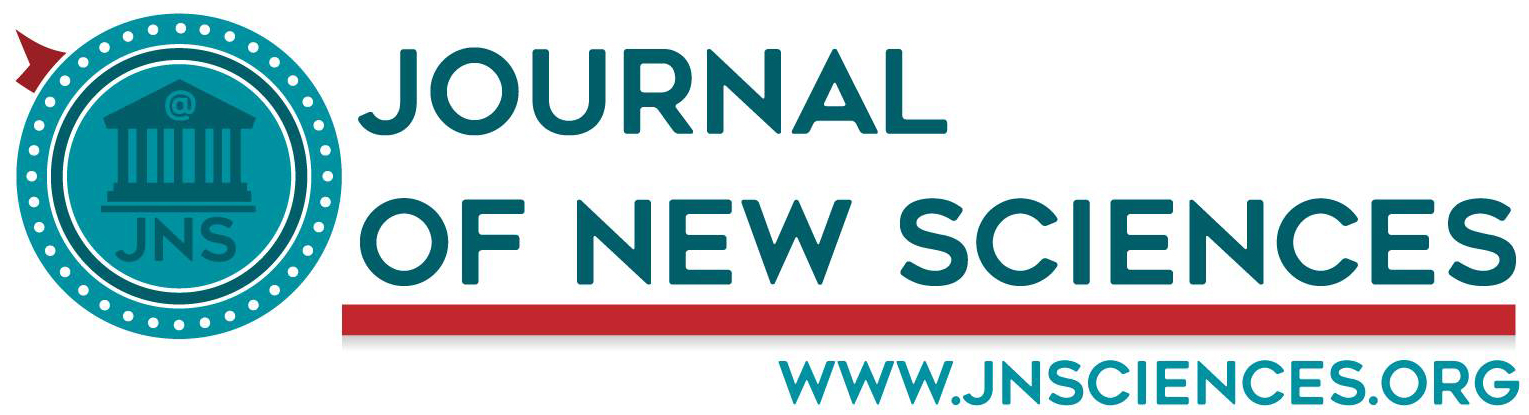
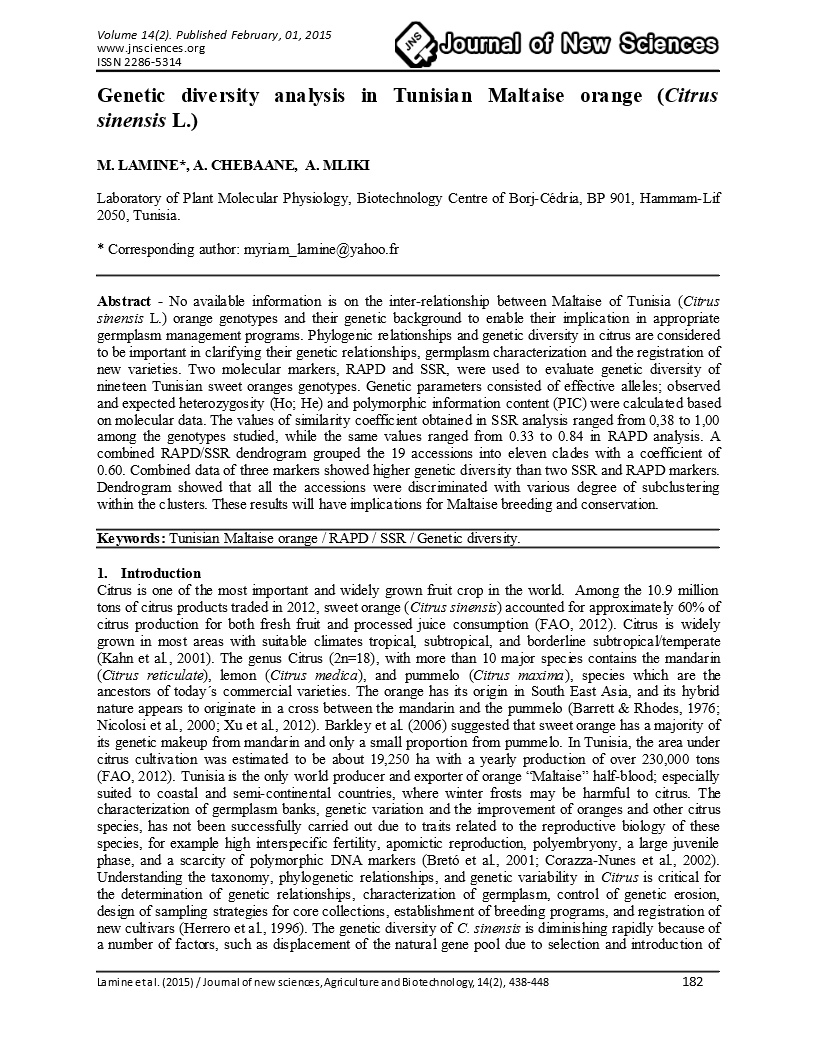
- Category: Volume 14
- Hits: 9485
Genetic diversity analysis in Tunisian Maltaise orange (Citrus sinensis L.)
M. Lamine*
A. Chebaane
A. Mliki
Laboratory of Plant Molecular Physiology, Biotechnology Centre of Borj-Cédria, BP 901, Hammam-Lif 2050, Tunisia.
Abstract - No available information is on the inter-relationship between Maltaise of Tunisia (Citrus sinensis L.) orange genotypes and their genetic background to enable their implication in appropriate germplasm management programs. Phylogenic relationships and genetic diversity in citrus are considered to be important in clarifying their genetic relationships, germplasm characterization and the registration of new varieties. Two molecular markers, RAPD and SSR, were used to evaluate genetic diversity of nineteen Tunisian sweet oranges genotypes. Genetic parameters consisted of effective alleles; observed and expected heterozygosity (Ho; He) and polymorphic information content (PIC) were calculated based on molecular data. The values of similarity coefficient obtained in SSR analysis ranged from 0,38 to 1,00 among the genotypes studied, while the same values ranged from 0.33 to 0.84 in RAPD analysis. A combined RAPD/SSR dendrogram grouped the 19 accessions into eleven clades with a coefficient of 0.60. Combined data of three markers showed higher genetic diversity than two SSR and RAPD markers. Dendrogram showed that all the accessions were discriminated with various degree of subclustering within the clusters. These results will have implications for Maltaise breeding and conservation.
Keywords: Tunisian Maltaise orange / RAPD / SSR / Genetic diversity.
-
Introduction
Citrus is one of the most important and widely grown fruit crop in the world. Among the 10.9 million tons of citrus products traded in 2012, sweet orange (Citrus sinensis) accounted for approximately 60% of citrus production for both fresh fruit and processed juice consumption (FAO, 2012). Citrus is widely grown in most areas with suitable climates tropical, subtropical, and borderline subtropical/temperate (Kahn et al., 2001). The genus Citrus (2n=18), with more than 10 major species contains the mandarin (Citrus reticulate), lemon (Citrus medica), and pummelo (Citrus maxima), species which are the ancestors of today´s commercial varieties. The orange has its origin in South East Asia, and its hybrid nature appears to originate in a cross between the mandarin and the pummelo (Barrett & Rhodes, 1976; Nicolosi et al., 2000; Xu et al., 2012). Barkley et al. (2006) suggested that sweet orange has a majority of its genetic makeup from mandarin and only a small proportion from pummelo. In Tunisia, the area under citrus cultivation was estimated to be about 19,250 ha with a yearly production of over 230,000 tons (FAO, 2012). Tunisia is the only world producer and exporter of orange “Maltaise” half-blood; especially suited to coastal and semi-continental countries, where winter frosts may be harmful to citrus. The characterization of germplasm banks, genetic variation and the improvement of oranges and other citrus species, has not been successfully carried out due to traits related to the reproductive biology of these species, for example high interspecific fertility, apomictic reproduction, polyembryony, a large juvenile phase, and a scarcity of polymorphic DNA markers (Bretó et al., 2001; Corazza-Nunes et al., 2002). Understanding the taxonomy, phylogenetic relationships, and genetic variability in Citrus is critical for the determination of genetic relationships, characterization of germplasm, control of genetic erosion, design of sampling strategies for core collections, establishment of breeding programs, and registration of new cultivars (Herrero et al., 1996). The genetic diversity of C. sinensis is diminishing rapidly because of a number of factors, such as displacement of the natural gene pool due to selection and introduction of genotypes suitable for intensive horticulture forming a limited gene pool (Agora, 2000). DNA markers are being widely used in studying polymorphism between species or in populations. In Citrus, PCR-based markers have been used for genetic mapping (Cai et al., 1994), to study genetic relationship among species or cultivars ( Luro et al., 1995; Coletta-Filho et al., 1998; Abkenar and Isshiki, 2003) to discriminate Citrus hybrids (Elisiario et al., 1999) and to identify Citrus mutants (Deng et al., 1995) and periclinal chimeras (Sugawara et al., 2002). RAPD markers that result from the PCR amplification of genomic DNA fragments using short oligonucleotide of arbitrary sequence as primers (William et al., 1990) provide a speedy and easy approach for taxonomic classification and cultivar-typing of fruit trees. This assay has the advantage of being readily employed, requiring very small amounts of genomic DNA, and eliminating the need for blotting and radio-active detection (Cipriani et al., 1996). Factors such as speed, efficiency and amenability to automation make it one of the most appropriate methods for germplasm management with respect to estimating diversity, monitoring genetic erosion and removing duplicates from germplasm collections (Khadari et al., 2003). Besides of RAPD, simple sequence repeats (SSRs) has been also used in the analysis of genetic diversity of many plant species such as apple (Guilford et al., 1997) and grape (Thomas and Scott, 1993). Citrus microsatellites have been used for different purposes (Barkley et al. 2006; Luro et al., 2008; Jannati et al., 2009; Ollitrault et al. 2010) such as screening of zygotic seedlings among nucellar seedling population (Oliveira et al. 2002) and germplasm characterization (Jiang et al. 2006; Novelli et al. 2006). The present study aims to evaluate the genetic variation within Maltaise orange genotypes cultivated either commercially or traditionally in Tunisia, as well as to investigate their inter-relationships by using RAPD and SSR ‘fingerprint’.
-
Materials and methods
2.1. Plant materials
A total of 19 Maltaise orange (C. sinensis L.) genotypes (Table 1) were investigated in the present study. Leaf samples were collected from the citrus orchards located at Cap Bon region in North East of Tunisia.
Table 1. Tunisian Maltaise genotypes used for molecular marker |
|||
AnalysisCode |
Genotypes |
Site |
Origin |
A1 |
Sotam 8 |
Hchicha |
Takelsa |
A2 |
SP 18 |
Ben hachem |
Bni khalled |
A3 |
Sotam 2 |
Hchicha |
Takelsa |
A4 |
F 19 |
Ghars mrad |
Chrifet |
A5 |
H20 |
Béchir |
Chrifet |
A6 |
F 7 |
Ahmed |
Chrifet |
A7 |
H 8 |
Tarak bekir |
Chrifet |
A8 |
M 5 |
Béchir |
Mrayssa |
A9 |
SM 1 |
Bchira |
Bni khalled |
A10 |
M 1 |
Béchir |
Mrayssa |
A11 |
F 10 |
Ahmed |
Chrifet |
A12 |
F 25 |
Ahmed |
Chrifet |
A13 |
Sotam 7 |
Hchicha |
Takelsa |
A14 |
H 16 |
Tarak bekir |
Chrifet |
A15 |
F 5 |
Gaddour |
Chrifet |
A16 |
F 39 |
Ahmed |
Chrifet |
A17 |
SP 9 |
Ben hachem |
Bni khalled |
A18 |
S 1 |
Bchira |
Bni khalled |
A19 |
Sotam 3 |
Hchicha |
Takelsa |
2.2. DNA extraction
The isolated leaf material was used for genomic DNA extraction by using a citrus specific protocol. Leaf samples were ground with liquid nitrogen in separate 2 ml eppendorf tubes. Extraction buffer [100 mM Tris-Hcl, 50 mM EDTA, 500 mM NaCl, SDS (20%) and 140 mM B-mercaptoethanol] was added, vortexed and incubated at 65°C for 30 min. After that, potassium acetate (5M) was added in each tube and the mixture was incubated on ice for 30 min. Subsequently, all tubes were centrifuged at 12000 rpm for 30 min. Then, isopropanol was added to the aqueous layer, the DNA was pelleted by centrifugating for 20 min and washed with 80 % ethanol. The DNA was dissolved in TE buffer and quantified before a dilution step to obtain uniform concentration of 50 µg/ml.
2.3. RAPD analysis
Table 2. List of the RAPD primers used for the investigation of genetic diversity within Maltaise orange genotypes. |
|||
Primer name |
Total amplified bands |
Polymorphic bands |
% of polymorphism |
OPA-01 |
14 |
10 |
71.0 |
OPA-04 |
21 |
21 |
94.0 |
OPA-05 |
15 |
12 |
43.0 |
OPA-08 |
16 |
16 |
60.8 |
OPA-09 |
11 |
11 |
69.8 |
OPA-11 |
22 |
22 |
95.0 |
OPA-14 |
11 |
8 |
66.0 |
OPB-02 |
15 |
12 |
94.4 |
OPB-10 |
16 |
14 |
60.0 |
OPC-05 |
17 |
16 |
90.0 |
OPC-06 |
19 |
18 |
94.0 |
OPC-11 |
18 |
14 |
60.0 |
Total |
195 |
174 |
89.8 |
Twelve decamer primers were used for PCR reaction (Table 2). PCR was carried out in a 15 µl reaction mixture containing 25-50 ng total DNA, 0.8 µM primer, 2.0 mM MgCl2, 200 µM dNTPs, 10 x Buffer and 1U Taq-DNA polymerase. PCR conditions were 3 min at 93°C followed by 40 cycles, each consisting of 1 min at 93°C, 1 min at 37°C, and 2 min at 72°C. The amplification products were separated by electrophoresis on 1.5% agarose gel in TAE buffer.
2.4. SSR analysis
Nine markers (Table 3) presented proper and clear results (Kijas et al.1997; Froelicher et al. 2008; Luro et al. 2008) were used for the diversity study. PCR was performed in a final volume of 15 µl. Each PCR reaction consisted of 1,5 mM of MgCl2, 2,5 mM of dNTPs, 0,2 µM of each primer and 1U of DNA Taq polymerase with 50 ng of DNA. Cycling conditions were: 94°C for 5 min as an initial denaturation step before entering 40 cycles each composed of 30 sec at 94°C, 30 sec at annealing temperature, 1 min at 72°C and a final extension step of 4 min at 72°C.
Table 3. Polymorphism information for SSR markers used to evaluate genetic diversity among maltaise accessions.
|
||||||
Primers |
Allele number |
Genotype number |
He |
Ho |
Gene diversity |
Pic |
TAA1 |
3 |
16 |
0.5391 |
0.0000 |
0.575 |
0,539 |
TAA27 |
2 |
15 |
0.1244 |
0.0000 |
0.133 |
0,125 |
GT03 |
2 |
19 |
0.4321 |
0.6316 |
0.439 |
0,433 |
CT02 |
2 |
18 |
0.4012 |
0.5556 |
0.408 |
0,41 |
CAG01 |
2 |
19 |
0.4654 |
0.7368 |
0.471 |
0,467 |
CAT01 |
1 |
19 |
0.0000 |
0.0000 |
0.000 |
0.000 |
ATC09 |
3 |
19 |
0.5831 |
1.0000 |
0.588 |
0,584 |
CT21 |
2 |
18 |
0.0540 |
0.0556 |
0.056 |
0,049 |
CAGG9 |
2 |
19 |
0.5000 |
1.0000 |
0.500 |
0.500 |
Total |
10 |
94 |
|
|
|
|
Mean |
2.11 |
|
0,344 |
0,442 |
|
0,3165 |
2.5. Statistical analysis
The patterns at all RAPD loci were scored for each polymorphic band as 1 for band presence and 0 for band absence. Scores were entered into a binary matrix on the subprogram NTedit of NTSYSpc v.2.10e program (Rohlf, 2002). The data were analyzed with module SIMQUAL to generate genetic similarity coefficients and then ordered in a similarity matrix (Jaccard, 1908). The similarity matrix was run on module SAHN (sequential, agglomerative, hierarchical, and nested) (Sneath and Sokal, 1973) by using the Unweighted Pair group method with arithmetic average (UPGMA) clustering algorithm (Sokal and Michener, 1958) to generate a dendrogram. For SSRs, GENETIX 4.02 computer package (Belkhir, 1999) was used to calculate gene diversity per locus, expected heterozygosity (He; Nei, 1973) and observed heterozygosity (Ho) over 9 loci. Polymorphism information content (PIC) values were calculated, for RAPD and SSR loci, according to Smith et al. (1997), using the algorithm for all primer combinations as follows: PIC =1 – , where
is the frequency of the ith allele. PIC provides an estimate of the discriminatory power of a locus by taking into account, not only the number of alleles that are expressed but also the relative frequencies of those alleles. Principal Coordinate analysis (PCA) was performed to identify accession groups using XLSTAT software. In this procedure, the similarity matrix was used to generate Eigen values and scores for the accessions. This multivariate approach was chosen to complement the cluster analysis information; because cluster analysis is more sensitive to closely related individuals, while, the PCO is more informative regarding distances among major groups.
3. Results
3.1. RAPD analysis
Twelve primers (Table 2) were selected for the RAPD analysis based on the reproducibility and banding patterns. PCR amplification of the genomic DNA isolated from 19 cultivars of Maltaise orange (C. sinensis) yielded a total of 195 bands, of which 174 were polymorphic (Table 2). The total number of amplified DNA bands ranged from 11 (OPA09 and OPA14) to 22 (OPA11), with an average of 16.25 bands per primer. The polymorphism percentage ranged from 43 (primer OPA05) to 95% (OPA11) with an average of 74.83 %. The pair-wise similarity values obtained between 19 Maltaise cultivars ranged from 0.333 (between A9 and A10) to 0.845 (between A11 and A12). The average similarity across all the cultivars was 0.58. A dendrogram was constructed using UPGMA method cluster analysis on the basis of Jaccard’s coefficients grouped the 19 cultivars into three major clusters (Figure 1). The first cluster was the biggest comprising 17 cultivars. Within this cluster, the cultivars A11 and A12 were genetically most similar, showing 84.5% of genetic similarity. The second cluster consisting of the cultivars A10 and A16; while the cultivars A9 was individually separated into distinct group from the rest of cultivars.
|
Figure 1. Dendrogram illustrating the phylogenetic relationship among the nineteen Maltaise genotypes based on RAPD markers. |
3.2. SSR analysis
The polymorphism level of the 9 SSR loci was investigated in all 19 citrus accessions. The number of putative alleles per locus ranged from 1 (CAGG9) to 3 (ATC09, GT03) with an average of 2.11 alleles per locus (Table 3). The observed heterozygosity (Ho) ranged from 0.00 (ATC09, CAG01 and CAGG9) to 1.00 (GT03, TAA27) with a mean of 0.44. The expected heterozygosity (He) ranged from 0.00 (CAGG9) to 0.583 (GT03) with a mean of 0.344. GT03 was the most informative marker and CAGG9 was the least informative marker. The PIC values for the 9 markers ranged from 0.000 (CAGG9) to 0.584 (GT03) with a mean of 0.372. The cluster analysis separated all the accessions, at similarity index of 0.694, into four main clusters (Figure 2).
|
Figure 2. Dendrogram illustrating the phylogenetic relationship among the nineteen Maltaise genotypes based on SSR markers. |
3.3. Genetic diversity displayed by RAPD and SSRs
In order to find out the genetic relationship between different sweet orange accessions, SSR and RAPD data sets were combined together for analysis. The Jaccard’s similarity coefficient, for the combined data, ranged from 0.36 to 0.84 with an average of 0.60 among all the 19 accessions used.
|
Figure 3. Dendrogram illustrating the phylogenetic relationship among the Maltaise genotypes based on combined RAPD and SSR markers. |
The genetic relationship between the accessions was clearly depicted in the dendrogram (Figure 3) and grouped the accessions in eleven main clusters. Within the first cluster, two subgroups consisting of four genotypes were observed. The second and third cluster consisted of 3 and 4 genotypes, respectively. The other remaining genotypes (8) were differentiated and formed various degree of sub-clustering. There was no relationship between the spatial and genetic proximity of the germplasm. The Mantel test (Figure 4) performed among the genetic distance matrices obtained from RAPD and SSR analyses, showed a significant correlation (p<0.05) between RAPD and SSR (R=0.272) which indicated good concordance between the clusters that were suggested by the two data sets.
|
Figure 4. Regression plot revealing between RAPD and SSR markers matrices across the studied genotypes. |
PCA is more adapted than tree representation in describing the organization of genetic diversity when hybrids between differentiated groups are frequent in the sample. Data generated from SSR and RAPD genotyping of 19 maltaise cultivars were subjected to Principle Coordinates Analysis (PCA) (Figure 5). The first two axes explain 31.10% of the total variance. Roughly, cultivar plotting is independent of geographic origin and Maltaise accessions from all regions were broadly overlapped.
-
Discussion
Our results showed that out of 195 bands, 90% among them were polymorphic (Table 1) which represents the most important polymorphism proportion compared to other previous reports on Citrus genetic diversity. In fact, 65% polymorphism was found in 37 Citrus varieties in Vietnam (Nhan et al., 2003), 39% of polymorphic bands obtained in 33 Volkamerian lemon in Mexico (Andrade-Rodriguez et al., 2004) and 51.51% polymorphism was detected within 22 sweet orange accessions in India (Malik et al., 2012). The level of polymorphism of the same primer may depend on both conditions and varieties, for example, using OPA04 primer, we found a very high level of polymorphism (94 %) within Tunisian sweet orange, while, no polymorphism was recorded (0 %) among 22 Indian sweet orange (Malik et al., 2012). Pair-wise similarity analysis of 12 RAPD markers in the 19 cultivars of C. sinensis revealed a wide genetic background for Tunisian sweet orange. Citrus is almost universally propagated by budding scion cultivars onto apomictic seedling rootstocks (Elisiario et al., 1999) leading to a high level of genetic uniformity either in the same plantation (Fang and Roose, 1997; Elisiario et al., 1999). However, in our study, the Maltaise genotypes investigated did not cluster according to the geographical origin, since we observed a high level of genetic polymorphism among them. That could be explained by the occurrence of a high rate of cross pollination influenced by the relatively-limited distribution area of this species. With respect to DUS (distinctness, uniformity and stability) criteria for cultivar identification, the use of RAPD bands for distinguishable inter-varietal variation could be of genetic and agronomic importance. This would have an importance for the establishment of property rights and determination of variety purity. Such unique bandings would be especially valuable if they are linked to agronomically important traits.
The efficiency of 9 SSR markers for the characterization of sweet orange has been tested and evaluated using SSR markers in citrus (Novelli et al., 2006). Considering all the 9 SSR markers used in the present study, a relatively low polymorphism was detected across the analyzed germplasm. In terms of alleles' number, all the markers could detect a relatively low number of alleles (19 alleles), with an average of 2.11 per locus (Table 2). This result was similar to that found by Valdenice et al. (2006) when studying sweet oranges. Recently, Pireseyedi et al. (2010) found similar results (2.9 alleles per locus) across 60 Iranian pomegranate accessions using 12 SSR markers. Such as low number of detected alleles is often found in cultivated fruit species, especially those vegetatively propagated, fig (Giraldo et al., 2005), and peach (Cheng and Huang, 2009), while a higher number of alleles could be found in other perennial species, including olive (Carriero et al., 2002) and lychee (Viruel and Hormaza, 2004). Polymorphic information content (PIC) is a measure of a marker’s informativeness. The PIC values for the 9 markers ranged from 0 (CAGG9) to 0.58 (GT03) with a mean of 0.37, which was lower to that found in previous study (Golein et al., 2012). The overall heterozygosity calculated on the studied set of Maltaise cultivars is found to be quite low (Table 3). Self-pollination, followed by vegetative propagation in production, may have shaped the current diversity structure in the citrus germplasm. The relatively low level of diversity detected by microsatellite markers reflects a narrow genetic background of the local studied germplasm in Tunisia. This result was described in previous studies (Luro et al., 2001). The low intraspecific diversity found in cultivated species such as sweet orange contrasts with the high variability of agriculturally important traits such as ripening period and color and size of fruits (Bretó et al., 2001). Because of these factors and the general lack of citrus molecular markers the distinction between cultivars is still based mainly on morphological traits, especially fruit traits (Fang and Roose, 1997). The data of the present study is comparable with others (Barkley et al., 2006; Uzun et al., 2009) which reported there is close molecular relationship among sweet oranges. The sweet orange genetic resources in Tunisia apparently have been subjected to human selection for centuries. Therefore, only a small part of genome, coding for fruit traits (agronomic characters), is involved. These results suggest that the cultivated citrus germplasm in Tunisia, which is considered as diversification center of this crop, may share a common genetic bottleneck. The polymorphic SSR loci allowed the detection of three synonymous groups involving 8 accessions (Figure 2), suggesting a substantial amount of genetic redundancy in the Tunisian maltaise collection. The case of this high level of redundancy is a common problem in many plant germplasm collections (Engels and Visser, 2003; Zhang et al., 2009), which impede the accuracy and efficiency of conservation and utilization of plant genetic resources. It is known that the there has been extensive exchange of maltaise oranges planting material (cuttings) in Tunisia, both among farms and among regions. Cultivars were often named according to the places where the cuttings were taken. This practice of cutting introduction can easily lead to duplicates in the germplasm collection. According to Luro et al. (2000) microsatellites could be considered a useful maker for citrus taxonomic studies but not so powerful for cultivar identification in citrus or other species where mutations or human selections are the basic mechanism for intra-specific differentiation. Difficulties in obtaining markers for the characterization of sweet oranges was reported by Orford et al. (1995) who identified no differences among sweet orange cultivars analyzed using minisatellite markers and similar results were observed by Novelli et al. (2000) using isozymes and RAPD markers (Targon et al., 2000).
The data obtained from RAPD and SSR were combined and phonetically analyzed to determine the genetic similarity among the accessions of the core collection. Each type of marker possesses distinct advantages and disadvantages, and it is more effective to use a combination of different marker systems to characterize genetic diversity in plants than a single system. Furthermore, the use of different types of molecular markers, which analyze different regions of the genome, permits a better and deeper analysis of genetic variations, as strongly recommended in several somaclonal variability studies (Hvarleva et al., 2008). RAPD primers quickly scan the whole genome detecting point mutations and insertion-deletion events (Milbourne et al., 1997). In fact, The dendrogram obtained with RAPD markers was different to that obtained with SSRs (Figure 1-2). When using both markers the dendrogram were divided into eleven cluster and all the accessions were well differentiated (Figure 3). Indeed, when all two sets of markers were combined into a single data set and analyzed together, the cophenetic correlation was 0.60, which is generally considered to be a high value (Rohlf, 2002). This indicates that a combination of the two marker systems gives a better estimate of similarity than any single marker type alone. The fingerprint of each genotype is defined by multiple RAPD and SSR bands presumably at multiple genetic loci. This is important for cultivar characterization since each cultivar is not defined by a single marker but by a set of several markers. This level of polymorphism probably reflects the outcrossing nature of citrus since similar results have been obtained using combination of SSR and RAPD markers in other crops (Mir et al.. 2008; Ebrahimi et al., 2011). Mantel test showed weak but significant, correlation among the RAPD and SSR similarity matrices (R = 0.272; p<0.05) and good concordance between the clusters that were suggested by the two data sets.
|
Figure 5. The plot of the two first components of PCA based on RAPD and SSR markers of the 19 Maltaise genotypes . |
Similar low correlation was obtained in soybean (Giancola et al., 2002) and sorghum (Geleta et al., 2006), while, high correlations between genetic distances obtained from different markers classes were also reported for fig (Salhi-Hannachi et al., 2005) and durum wheat (Maccaferri et al., 2007). The quite narrow scattering area of Maltaise cultivars on the PCA plot (Figure 5) can be explained by the decreased interest in cultivating Maltaise trees in the cap bon regions, since several decades, in aid of other commercially interesting crops (mainly grapevine). Besides, at regional level, results of the present study indicate that there is no discernable genetic difference within each region as well as among regions. The reason might be associated with dominant or co-dominant nature of the two markers and the different types of polymorphism detected (Belaj et al., 2003, Scariot et al., 2007).
4. Conclusion
The results in this study illustrate the combined efficiency of the SSR and RAPD techniques in discriminating the Maltaise genotypes analyzed. The significance of this bifurcation is currently unknown. But the current findings can be exploited in future once these findings will be correlated with traits of interest. Our report is the first to describe genetic diversity within Tunisian Maltaise orange germplasm. The combination of SSR and RAPD marker methods also guarantees some additional benefits and seem to be suitable for the finely tuned identification of tightly linked Maltaise genotypes, indicating their usefulness for an accurate identification of plants with tight genetic relations, and the results presented can form the basis for the design of future Citrus genetic improvement projects.
5. References
Abkenar AA and Isshiki S (2003) Molecular characterization and genetic diversity among Japanese acid citrus (Citrus spp.) based on RAPD markers. J. Hort Sci. Biotech. 78(1), 108-112.
Andrade-Rodriguez M, Villegas-Monter A, Carillo-Castaneda G, Garcia-Velazquez A (2004) Polyembriony and identification of Volkamerian lemon zygotic and nucellar seedlings using RAPD. Pesq. agropec. bras. 39, 551-559.
Agora R.K (2000) In situ conservation of biological diversity in Citrus. In: Proceedings of the Global Citrus Germplasm Network, app. 7, GCGN Meeting, 7–8 Dec.
Barkley N.A, Roose ML, Krueger RR, Federici CT (2006) Assessing genetic diversity and population structure in a citrus germplasm collection utilizing simple sequence repeat markers (SSRs). Theor. Appl. Genet. 112, 1519-1531.
Belaj A et al. (2003) Comparative study of the discriminating capacity of RAPD, AFLP and SSR markers and of their effectiveness in establishing genetic relationships in olive. Theor. Appl. Genet. 107, 736–744.
Belkhir K, Borsa P, Goudet J, Chikhi L, Bonhomme F (2002) GENETIX v. 4.03, Logiciel sous Windows pour la ge´ne´tique des populations. Laboratoire Ge´nome et Population, Universite´ de Montpellier 2, Montpellier, France. http://www.univ-montp2.fr.
Breto MP, Ruiz C, Pina JA, Asins M.J (2001) The diversification of Citrus clementina Hort. ex Tan., a vegetatively propagated crop species. Mol Phylogenet Evol. 21:285–293.
Cai Q, Guy C.L, Moore G.A (1994) Extension of the linkage map in citrus using random amplified polymorphic DNA (RAPD) markers and RFLP mapping of cold-acclimation responsive loci. Theor. Appl. Genet. 89, 606-614.
Carriero F, Fontanazza G, Cellini F, Giorio G (2002) Identification of simple sequence repeats (SSRs) in olive (Olea europaea L.). Theor. Appl. Genet. 104, 301-307.
Cheng Z, Huang H (2009) SSR fingerprinting Chinese peach cultivars and landraces (Prunus persica) and analysis of their genetic relationship. Sci. Hortic. 120, 188–193.
Cipriani G, Di Bella R, Testolin R (1996) Screening RAPD primers for molecular taxonomy and cultivar fingerprinting in the genus Actinidia. Euphytica. 90, 169-74.
Coletta Filho HD, Machado M.A, Targon ML, Moreira M.C, Pompeu JR (1998) Analysis of the genetic diversity among mandarins (Citrus spp.) using RAPD markers. Euphytica, 102, 133–139.
Corazza-Nunes MJ, Machado MA, Nunes WMC, Cristofani M, Targon MLPN (2002) Assessment of genetic variability in grapefruits (Citrus paradisi Macf.) and pummelos (C. maxima Burm.Merr.) using RAPD and SSR markers. Euphytica. 126,169–176.
Deng ZN, Gentile A, Nicoloci E, Domina F, Vardi A, Tribulato E (1995) Identification of in vitro and in vivo lemon mutants by RAPD marker. J. Hort. Sci. 70, 117-125.
Ebrahimi S, Sayed-Tabatabaei BE, Sharifnabi B (2010) Microsatellite isolation and characterization in pomegranate (Punica granatum L.). Iran. J. Biotechnol. 8, 156–163.
Ebrahimi A, Fatahi R, Zamani Z (2011) Analysis of genetic diversity among some Persian walnut genotypes (Juglans regia L.) using morphological traits and SSRs markers Scientia. Sci Hortic. 130, 146–151.
Elisiario P.J, Justo E.M, Leitão JM (1999) Identification of mandarin hybrids by isozyme and RAPD analysis. Sci Hort. 8, 287-299.
Engels JMM, Visser L (2003) A guide to Effective Management of Germplasm Collections.
Fang D, Roose M, Krueger R, Federici C (1997) Fingerprinting trifoliate orange germplasm accessions with isozymes, RFLPs, and inter-simple sequence repeat markers. Theor. Appl. Genet. 95, 211-219.
FAO (2012) Food and Agricultural Organization of the United Nations. Available at [http://www.fao.org].
Froelicher Y, Dambier D, Costantino G, Lotfy S, Didout C, Beaumont,V, Brottier P, Risterucci AM, Luro F, Ollitrault P (2008) Characterization of microsatellite markers in Citrus reticulate Blanco. Mol Ecol Resour. 8, 119–122.
Geleta N, Labuschagne MT, Viljoen CD (2006) Genetic diversity analysis in sorghum germplasm as estimated by AFLP, SSR and morpho-agronomical markers. Biodivers. Conserv. 15, 3251–3265.
Giancola S, Marcucci Poltri S, Lacaze P, Hopp HE (2002) Feasibility of integration of molecular markers and morphological descriptors in a real case study of a plant variety protection system for soybean. Euphytica 127, 95–113.
Giraldo E, Viruel M.A, López-Corrales M, Hormaza JI (2005) Characterisation and cross-species transferability of microsatellites in the common fig (Ficus carica L.) J. Hort. Sci. Biotechnol. 80, 217–224.
Golein B, Nazeryan M, Babak B (2012) Assessing genetic variability in male sterile and low fertile citrus cultivars utilizing simple sequence repeat markers (SSRs). African Journal of Biotechnology. 11(7), 1632-1638.
Guilford P, Prakash S, Zhu JM, Rikkerink E, Gardiner S, Bassett, H, Forster R (1997) Microsatellites in Malus×domestica (apple): Abundance, polymorphism and cultivar identification. Theor. Appl. Genet. 94, 249–254.
Hvarleva T, Kapari-Isaia T, Papayiannis L, Atanassov A (2008) Characterization of Citrus cultivars and clones in Cyprus through microsatellite and RAPD analysis. Biotechnol. Biotec. Eq. 22, 787-794.
Herrero R, Asíns MJ, Carbonell EA, Navarro L (1996) Genetic diversity in the orange subfamily Aurantioideae. I. Intraspecies and intragenus genetic variability. Theor. Appl. Genet. 92, 599-609.
Jannati M, Fotouhi R, Pourjan Abad A, Salehi Z (2009) Genetic diversity analysis of Iranian citrus varieties using micro satellite (SSR) based markers. Journal of Horticulture and Forestry. 1(7), 120-125.
Jiang D, Zhong GY, Hong QB (2006) Analysis of microsatellites in citrus unigenes. Acta Genetica Sinica. 33, 345–353.
Kahn TL, Krueger RR, Gumpf DJ, Roose ML, Arpaia ML, Batkin TA, Bash JA, Bier OJ, Clegg MT, Cockerham ST (2001) Citrus genetic resources in California: Analysis and recommendations for long-term conservation. Report No. 22. University of California Division of Agriculture and Natural Resources, Genetic Resources Conservation Program, Davis CA, USA.
Khadari B, Hochu I, Santoni S (2003) Which molecular markers are best suited to identify fig cultivars: a comparison of RAPD, ISSR and microsatellite markers. Acta Hortic. 605, 69-89.
Kijas JMH, Thomas MR, Fowler JCS, Roose ML (1997) Integration of trinucleotide microsatellites into a linkage map of citrus. Theor Appl Genet. 94, 701–706.
Luro F, Laigret F, Bové JM, Ollitraut P (1995) DNA amplified fingerprinting, a useful tool for determination of genetic origin and diversity analysis in citrus. Hort science. 30 (5), 1063-1067.
Luro F, Rist D, Ollitrault P (2000) Sequence tagged microsatellites polymorphism: an alternative tool for cultivar identification and evaluation of genetic relationships in Citrus. Proc. Int. Soc. Citricult. IX. Congr. Florida, USA. pp: 170–171.
Luro F, Rist D, Ollitrault P (2001) Evaluation of genetic relationships in Citrus Genus by means of Sequence Tagged Microsatellites. Proceedings of the International Symposium on Molecular Markers for Characterizing Genotypes and Identifying Cultivars in Horticulture February 2001; Montpellier, France. Acta Hortic. 546, 237-242.
Luro F, Costantino G, Terol J, Argout X, Allario T, Wincker P (2008) Transferability of the EST-SSRs developed on Nules clementine (Citrus clementina Hort ex Tan) to other Citrus species and their effectiveness for genetic mapping. BMC Genomics. 9, 287.
Maccaferri M, Stefanelli S, Rotondo F, Tuberosa R, Sanguineti MC (2007) Relationships among durum wheat accessions. I. Comparative analysis of SSR, AFLP, and phenotypic data. Genome 50, 373–384.
Malik SK, Rohini MR, Susheel K, Ravish C, Digvender P, Rekha C (2012) Assessment of Genetic Diversity in Sweet Orange [Citrus sinensis (L.) Osbeck] Cultivars of India using Morphological and RAPD Markers. Agric Res. DOI 10.1007/s40003-012-0045-3.
Mantel N (1967) The detection of disease clustering and a generalized regression approach. Cancer Res. 27, 209–220.
Milbourne D, Meyer R, Bradshaw JE, Baird E, Bonar N, Provan J, Powell W, Waugh R (1997) Comparison of PCR-based marker systems for the analysis of genetic relationships in cultivated potato. Mol Breed. 3, 127–136.
Mir JI, Karmakar P, Chattopadhyay S, Chaudhury SK, Ghosh SK, Roy A (2008) A Grouping of jute germplasm based on trait for fibre fineness through RAPD and SSR profiling and identification of contrasting parents—a prerequisite for mapping population development. In: Proceedings of International symposium on Jute and Allied Fibres Production, Utilization and Marketing, Kolkata, India. pp 26.
Nei M (1973) Analysis of gene diversity in subdivided populations. Proceedings of the National Academy of Sciences, USA 70, 3321-3323.
Nhan NT, Shimizu T, Hirohisa N, Omura M, Chau NM (2003) RAPD Markers: Application to varietal identification and analysis of genetic relationships among citrus varieties/species in Vietnam. Proceedings of the Annual Workshop of JIRCAS Mekong Delta Project, November 26-28, 2002, Faculty of Agriculture, Cantho University, Cantho, pp 1-8.
Nicolosi E, Deng ZN, Gentile A, La Malfa S (2000) Citrus phylogeny and genetic origin of important species as investigated by molecular markers. Theor. Appl. Genet. 100, 1155-1166.
Novelli VM, Machado MA, Romero Lopes C (2000) Isoenzymatic polymorphism in Citrus spp. and Poncirus trifoliata (L.) Raf. (Rutaceae). Genet. Mol. Biol. 23, 163-168.
Novelli VM, Cristofani M, Souza AA, Marcos A, Machado MA (2006) Development and characterization of polymorphic microsatellite markers for the sweet orange (Citrus sinensis L, Osbeck). Genet. Mol. Biol. 29, 90–96.
Oliveira AC, Garcia A, Cristofani M, Machado MA (2002) Identification of citrus hybrids through the combination of a leaf apex morphology and SSR markers. Euphytica 128(3), 397–403.
Ollitrault F., Terol J, Pina JA, Navarro L, Talon M, Ollitrault P (2010) Development of SSR markers from Citrus clementina (Rutaceae) BAC end sequences and interspecific transferability in Citrus. Am J Bot. 97, 124–129.
Orford SJ, Scot NS, Timmis JM (1995) A hypervariable middle repetitive DNA sequence from citrus. Theor Appl Genet. 91, 1248–1252.
Pireseyedi SM (2010) Isolation and characterization of novel microsatellite markers in pomegranate (Punica granatum L.). Int. J. Mol. Sci. 11, 2010–2016.
Rohlf FJ (2002) NTSYS-pc, Numerical Taxonomy and Multivariate Analysis System. Applied Biostatistics, New York.NTSYSPC. Numerical taxonomy system. Exter Software. New York.
Salhi-Hannachi A, Chatti K, Mars M, Marrakchi M, Trifi M (2005) Comparative analysis of genetic diversity in two Tunisian collections of fig cultivars based on random amplified polymorphic DNA and inter-simple sequence repeats fingerprints. Genet. Resour. Crop Evol. 52, 563–573.
Scariot V, De Keyser E, Handa T, De Riek J (2007) Comparative study of the discriminating capacity and effectiveness of AFLP, STMS and EST markers in assessing genetic relationships among evergreen azaleas. Plant Breeding. 126,207–212.
Smith JSC, Chin ECL, Shu H, Smith OS, Wall SJ, Senior ML, Mitchel SE, Kresorich S, Tiegle J (1997) An evaluation of the utility of SSR loci as molecular markers in maize (Zea mays): comparisons with data from RFLP and pedigree. Theor. Appl. Genet. 95, 163–173.
Sneath PHA, Sokal RR (1973) Numerical taxonomy. Freeman, San Francisco.
Sokal R, Michener C (1958) A statistical method for evaluating statistical relationships. Kans. Univ. Sci. Bul. 38, 1409-1438.
Sugawara K, Wakizuka T, Oowada A, Moriguchi T, Omura M (2002) Histogenic identification by RAPD analysis of leaves and fruit of newly synthesized chimeric Citrus. J. Am. Soc. Hortic. Sci. 127, 104-107.
Targon MLPN, Machado MA, Coletta Filho HD, Cristofani M (2000) Genetic polymorphism of sweet orange (Citrus sinensis L. Osbeck) varieties evaluated by random amplifi ed polymorphic DNA. Acta Hort. 535, 51-53.
Thomas MR, Scott NS (1993) Microsatellite repeats in grape- vine reveal DNA polymorphisms when analysed as sequence tagged sites (STSs). Theor. Appl. Genet. 86, 985– 990.
Uzun A, Yesilogu T, Aka-kacar Y, Tuzcu O, Gulsen O (2009) Genetic diversity and relationships within Citrus and related genera based on sequence related amplified polymorphism markers. Sci Hortic. 121, 306–312.
Valdenice MN, Mariangela C, Alessandra AS, Marcos AM (2006) Development and
characterization of polymorphic microsatellite markers for the sweet orange (Citrus sinensis L. Osbeck). Genet. Mol. Biol. 29, 90–96.
Viruel MA, Hormaza JI (2004) Development, characterization and variability of microsatellites in lychee ( Litchi chinensis Sonn., sapindaceae). Theor. Appl. Genet. 108, 896–902.
Williams JGK, Kubelik AR, Livak KJ, Rafalski JA, Tingey SV (1990) DNA polymorphisms amplified by arbitrary primers are useful as genetic markers. Nucleic Acids Research. 18, 6531- 6535.
Xu Q, Chen LL, Ruan X, Chen D, Zhu A, Chen C, Bertrand D (2012) The draft genome of sweet orange (Citrus sinensis). Nat. Genet. Doi: 10. 1038/ng.2472.