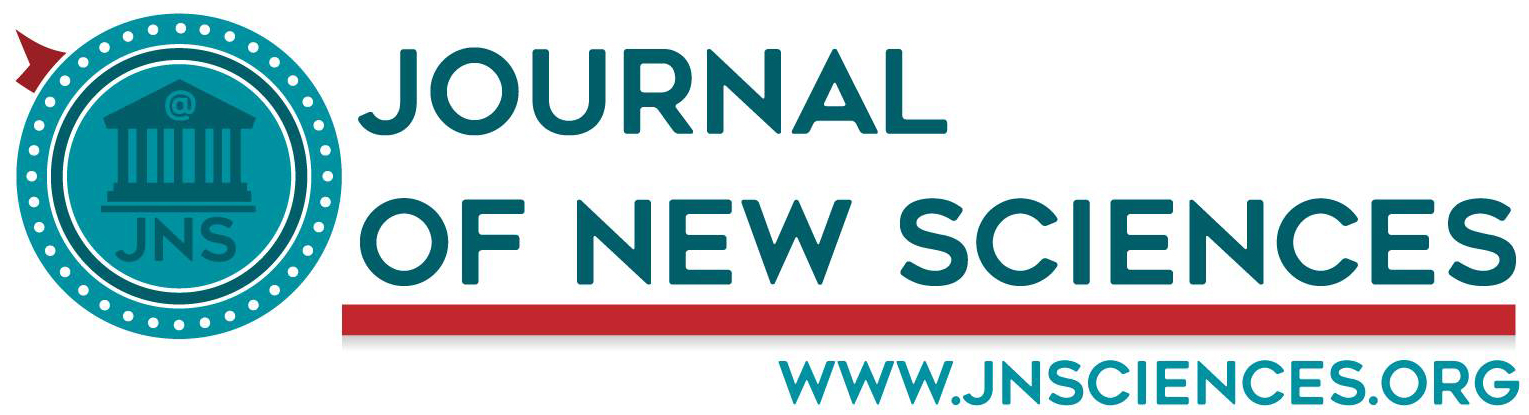
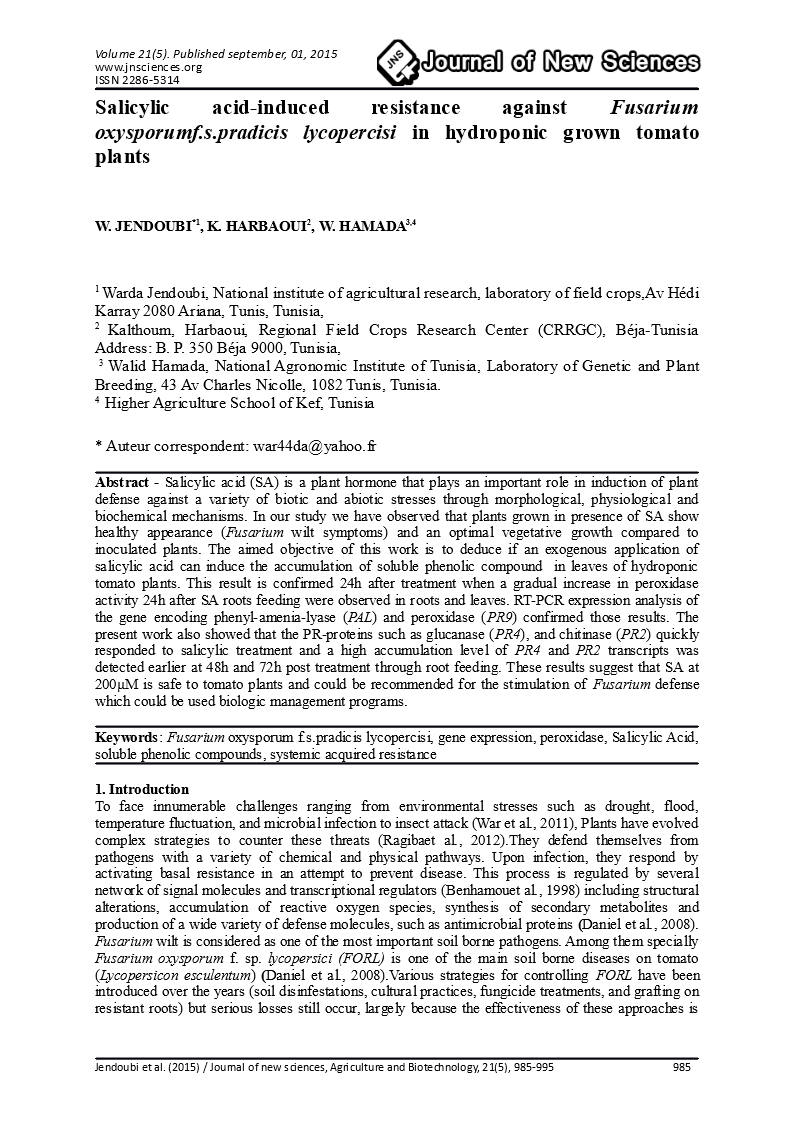
- Category: Volume 21
- Hits: 11588
Salicylic acid-induced resistance against Fusarium oxysporumf.s.pradicis lycopercisi in hydroponic grown tomato plants
W. Jendoubi*1
K. Harbaoui2
W. Hamada3,4
1 Warda Jendoubi, National institute of agricultural research, laboratory of field crops,Av Hédi Karray 2080 Ariana, Tunis, Tunisia,
2 Kalthoum, Harbaoui, Regional Field Crops Research Center (CRRGC), Béja-Tunisia Address: B. P. 350 Béja 9000, Tunisia,
3 Walid Hamada, National Agronomic Institute of Tunisia, Laboratory of Genetic and Plant Breeding, 43 Av Charles Nicolle, 1082 Tunis, Tunisia.
4 Higher Agriculture School of Kef, Tunisia
Abstract - Salicylic acid (SA) is a plant hormone that plays an important role in induction of plant defense against a variety of biotic and abiotic stresses through morphological, physiological and biochemical mechanisms. In our study we have observed that plants grown in presence of SA show healthy appearance (Fusarium wilt symptoms) and an optimal vegetative growth compared to inoculated plants. The aimed objective of this work is to deduce if an exogenous application of salicylic acid can induce the accumulation of soluble phenolic compound in leaves of hydroponic tomato plants. This result is confirmed 24h after treatment when a gradual increase in peroxidase activity 24h after SA roots feeding were observed in roots and leaves. RT-PCR expression analysis of the gene encoding phenyl-amenia-lyase (PAL) and peroxidase (PR9) confirmed those results. The present work also showed that the PR-proteins such as glucanase (PR4), and chitinase (PR2) quickly responded to salicylic treatment and a high accumulation level of PR4 and PR2 transcripts was detected earlier at 48h and 72h post treatment through root feeding. These results suggest that SA at 200µM is safe to tomato plants and could be recommended for the stimulation of Fusarium defense which could be used biologic management programs.
Keywords: Fusarium oxysporum f.s.pradicis lycopercisi, gene expression, peroxidase, Salicylic Acid, soluble phenolic compounds, systemic acquired resistance
1. Introduction
To face innumerable challenges ranging from environmental stresses such as drought, flood, temperature fluctuation, and microbial infection to insect attack (War et al., 2011), Plants have evolved complex strategies to counter these threats (Ragibaet al., 2012).They defend themselves from pathogens with a variety of chemical and physical pathways. Upon infection, they respond by activating basal resistance in an attempt to prevent disease. This process is regulated by several network of signal molecules and transcriptional regulators (Benhamouet al., 1998) including structural alterations, accumulation of reactive oxygen species, synthesis of secondary metabolites and production of a wide variety of defense molecules, such as antimicrobial proteins (Daniel et al., 2008). Fusarium wilt is considered as one of the most important soil borne pathogens. Among them specially Fusarium oxysporum f. sp. lycopersici (FORL) is one of the main soil borne diseases on tomato (Lycopersicon esculentum) (Daniel et al., 2008).Various strategies for controlling FORL have been introduced over the years (soil disinfestations, cultural practices, fungicide treatments, and grafting on resistant roots) but serious losses still occur, largely because the effectiveness of these approaches is variable and often short-lived. In addition, highly resistant tomato cultivars are not commercially available, although some breeding lines derived from cultivars Vender and Larma have proven to be good sources of resistance (Benhamou et al., 1994). Due to their destructive effects on many important crops, FORL has attracted great efforts in different research disciplines over the last century, aiming to develop effective control strategies (Ernesto et al., 1998). One of the main strategies consist in grafting commercial varieties on resistant rootstocks as Hymen or Bofor which reduces quality and yield of grafted plants. It is now well documented that treatment of plants with various agents used such as elicitors (e.g., virulent or avirulent pathogens, non-pathogens, cell wall fragments, plant extracts, and synthetic chemicals) can lead to the induction of resistance to subsequent pathogen attack exploiting both locally and systemically SAR (Walters et al., 2005). Elicitors refer to chemicals that can trigger physiological and morphological responses and secondary metabolism in plants (Benhamou, 1996). Elicitation causes a variety of defensive reactions and the accumulations of secondary metabolites (Yukimune et al., 1996; Zhang et al., 2004; Zhao et al., 2005).
Plant phytohormones such as ethylene, abscisic acid, jasmonic acid (JA), and salicylic acid (SA) are important components of different signaling pathways involved in plant defense. Exogenous application of SA and JA manipulates various physiological, biochemical and molecular processes in plants (war et al., 2011). Because SA plays a key role in a plant’s growth, development, and defense responses, and it is involved in some signal transduction systems to induce particular enzymes (Chen et al., 2006).
This work is devoted i) to study the expression of some protein related gene and ii) to determine the effect of SA treatment on phenol release, peroxidase activity and gene defense response in tomato (Lycopersicon esculentum), during Fusarium oxysporum f. sp.lycopersici infection, and after exogenous SA root feeding.
2. Material and Methods
2.1. Tomato plants and SA treatment
Tomato Seeds (Lycopersicon esculentum) Cv. ‘Riogrande’ highly susceptible to FORL were surface sterilized for 1 min in 75% ethanol and immerse for 3 min in sterile distilled water to germinate aseptically. The tomato seedlings were grown in hydroponic culture. Salicylic acid (Sigma) dissolved in distilled water was added to the MS liquid medium for root feeding at a final concentration of 200µM. Equal volume of distilled water was added to the hydroponic medium of control plants. In order to ensure a continuous supply of nutriments and required concentration of SA,the amended medium was renewed daily during one week as adopted by Sudhamony et al. (2009).
2.2. Pathogen inoculation
Fusarium oxysporum f.sp. radisislycopercisi strain, isolated from greenhouse tomato roots, was grown on potato dextrose agar medium in light at 20°C. Spores from 7-days-old cultures were removed gently from the surface of each plate culture by adding sterile distilled water. The spore suspension used for inoculation was applied at a concentration of 106 spores ml-1. Tomato plants were inoculated by the addition of the spore suspension in the hydroponic medium three days after the last SA application. No inoculated tomato plants were used as control.
2.3. Antifungal activity in vitro assay of SA against FORL
The direct effect of SA was tested in vitro by measuring the development of FORL myceliumon PDA medium amended with 30, 50, 100, 200, and 300µM SA solution. A plug (6 mm diameter) of fresh FORL mycelium was placed in the middle of the amended PDA plate. The mycelium growth was measured every three days until the total coverage of no SA amended PDA plate was reached.
2.4. Disease assessment
The assessment of disease severity was accomplished according to Vakalounakis and Fragkiadakis (1999). The inoculum used in this assay was prepared from microconidia grown during three to five days in shaking potato dextrose medium at 25°C. The concentration of conidia was assessed with Malassez cell evaluated and used to inoculate the seedlings at a final concentration of 106 spores ml-1. Two weeks after inoculation, the disease severity was recorded according to a visual scale from 0 to 3 (0: no symptoms; 1: light yellowing of leaves, light or moderate rot on tap root and secondary roots and crown rot; 2: moderate or severe yellowing of leaves with or without wilting, stunting, severe rot on tap root and secondary roots, crown rot with or without hypocotyls rot, and vascular discoloration in the stem; and 3: dead seedlings). Disease incidence was determined using the following formula (Song et al., 2004)
2.5. Extraction and quantification of soluble phenolic compounds
Levels of soluble phenolic compound were evaluated according to El Hadrami et al. (1997). Foliar tissue (200 mg) was homogenized in an ice bath with 1.5 ml methanol. The homogenate was centrifuged three times at 10,000 g for 5min, and the supernatants were recuperated each time. A volume of 100 µl of the supernatant was added to Folin-ciocalteu reagent and sodium carbonate. The mix was incubated at 40°C for 30 min and the blue color was read at 760nm. The content of soluble phenolic compounds was determined for the experiments described above and was expressed in mg-equivalents of Cathechin (Sigma) per g of fresh weight.
2.6. Peroxidase activity
Crude enzyme has been extracted with cold tris-maleate buffer 0.1 M, pH 6.5. The Peroxidase activity has been evaluated by spectrophotometer at 470 nm as described by EL Hadrami and Aauzac (1997) using Guaiacol (Sigma) as substrate.
2.7. RNA extraction and RT-PCR analysis
Leaves of SA treated plant were excised at 24h, 48h and 72h after SA application. Immersed in liquid nitrogen and stored at –80°C. Total RNAs were treated according to Trizol (Invitrogen) protocol. The reverse transcription reaction was carried out using MMLV-RT (Invitrogen) and subsequent cDNA was used as templates for PCR analysis. After the initial denaturing step at 94°C for 5 min, different cycles were performed. Each cycle consisted of denaturing step at 94°C for 30s annealing at 50°C for 30s, and elongation at 72°C for 30s with a final extension step performed at 72 °C for 7 min. The primers sequences used in PCR reactions are listed in table 1.
Table 1.Sequence of primers used in the RT-PCR reactions |
||
Gene |
Description |
Primers (Forward and Reverse) |
Tubilin |
Totub |
5′-CCAGGTTTGCCACTCACTTG-3′ 5′-GGAAAACGAAGAATGTGAGCAT-3 |
Chitinase |
PR4
|
5’-CTCCAATGGCTCTTCCACAT-3’ 5’-GAAATTGCTGCTTTCCTTGC-3’ |
Glucanase |
PR2
|
5’-TCTTGCCCCATTTCAAGTTC-3’ 5’-TGCACGTGTATCCCTCAAAA-3’ |
Peroxidase |
PR9
|
5’-GTCTCTTGTGCGGATATTGTTG-3’ 5’-ATTGTGGTGTTGTCATTGATGG-3’ |
protein isoform PR- P6 |
PR1
|
5’-ATAGTCTGGCCTCTCGGACA-3’ 5’-TCTTGTGAGGCCCAAAATTC-3’ |
Phenylalanine Ammonia-lyase |
PAL |
5’-GGTGTGAAGGCGAGTAGTG-3’ 5’-AGTTGAGGAGTGTTGTGATGG-3’ |
2.8. Statistical analysis
Data were analyzed by one-way analysis of variance (ANOVA) and Duncan’s multiple range tests using a statistical software package (SPSS 10.0 Inc.). There were five replications for each of the treatments.
3. Results
3.1. In vitro antifungal activity assay of SA against FORL
The mycelium growth of FORL was not significantly affected by SA added to PDA medium. None of the three tested concentrations of SA (30µM, 50µM, 100µM, 200µM, 300µM) was found to inhibit mycelia growth of FORL compared to the control.
3.2. Effect of SA treatment on disease incidence
The concentration of 200µM SA added to the hydroponic medium affected significantly the infection and the wilt development by FORL on tomato plants. The percentage of vascular browning and leaf yellowing wilting was remarkably reduced when plants were grown in presence of SA. Tomato plants inoculated with FORL, but not receiving SA treatment through roots, exhibited typical vascular browning and leaf yellowing wilting, while SA-treated plants showed less than 20℅ of vascular browning and leaf yellowing wilting only 2 weeks after inoculation (Figure 1).
|
Figure 1. Disease incidence of FORL on tomato hydroponic culture. Values with different letter are significantly different at α = 0.05. |
Two weeks after inoculation, SA-treated plants showed less than 9 ℅ of leaf yellowing compared to the control which exhibited disease incidence reaching 70 ℅. Moreover, plants treated three days before inoculation show healthy appearance and an optimal vegetative growth compared to inoculated plants. Applying SA 72h before inoculation has also a beneficial effect on root system development (Figure 2 B) compared to inoculated untreated plants (control) (Figure 2 A).
|
Figure 2. Effect of 200µM salicylic acid (SA) treatments72h before inoculation and FORL infestation on tomato seedlings grown in nutritive medium 15 days after inoculation. (A) Control inoculated plant exhibiting wilting; (B) plant treated with SA and inoculated 72 h after SA application of 200µM by root feeding. |
3.3. Effect of SA on soluble phenolic compounds
The addition of 200µM of SA to the hydroponic medium enhances the content of soluble phenols dices in the leaves 24h after application until 4 mg g-1 fresh weight. The value of 2.13 is higher than the amount found in the leaf of the untreated plants (Figure 3A). Content of free phenolic compounds is 4.7 times higher than those of control plants 72h after roots SA feeding. Similarly, this content is 2.48 times higher than the control at 48 h after root SA feeding (Figure 3A). The soluble phenolic content in leaves of treated infected plant showing no symptoms was 13.25 mg per g f wt, representing about 6 and 5.5 times higher than that found in untreated infected plants without disease symptoms respectively after 72h and 48h of inoculation (Figure 3 B). For all dates the released phenolic compound was higher in SA treated seedlings than the control.
|
Figure 3. Response of of phenolic content in tomato leaves to SA feeding during 72h. A:Time-course of changes in the content of free phenolic compounds in tomato leaves in response to 200µM SA. Each bar is the mean of three replicates of leaf extracts. B: Effect of SA treatments (200µM) on soluble phenolic contents in leafs of tomato plants 24h, 48h and 72h after inoculation with FORL. |
3.4. Effects of salicylic acid on the activities of PAL
RT-PCR expression analysis of the gene encoding phenyl-amenia-lyase (PAL) confirmed the results found earlier (Figure 4).The results showed that PAL transcripts were highly accumulated at 72h after SA roots feeding. This is correlated with a high levels of phenolic compounds measured in leaves at 72h.
|
Figure 4. expression patterns of PAL transcript accumulation analyzed by RT-PCR in tomato leaves after 24h (1), 48h (2) and 72h (3) of 200µM SA roots feeding compared to untreated plant (4) and to inoculated plants24h (5),48h (6) and 72h (7) after inoculation. Totub is the constitutive gene of tubulin in tomato used as positive control. |
3.5. Effects of salicylic acid on peroxidase activity
The treatment with 200µM SA have an effect on the peroxidase activity in roots and leaves of hydroponic tomato plants. Interestingly, the peroxidase activity of plants inoculated by FORL is 1.4 times higher in roots (Figure 5A) than in leaves (Figure 5B). Thus, the maximum increase in peroxidase activity has been reported at 72h post inoculation. Indeed, inoculation of control plants (+ FORL) induce peroxidase activity at 24 h post inoculation with 8.87 and 3.62 times higher than that of healthy plants (-SA) in roots and leaves respectively.
Peroxidase activity was 6.41 and 14.79 times higher in treated plants (+SA) at 72h in roots and leaves respectively. Peroxidase activity increased several fold in tomato leaves after 200µM SA feeding, indicating that the root system might have the capacity to assimilate and re-circulate SA throughout the plant or reduce the peroxidase activity by systemic signal in leaves.
|
Figure 5. Peroxidase activity detected in roots (A) and leaves (B) of tomato plants grown in liquid nutritive medium amended with SA and inoculated with FORL compared to the controls. |
3.6. Effects of salicylic acid on the expression of PR9 gene
To confirm the biochemical assay of peroxidase activity, a molecular study of the gene involved in the expression of peroxidase (PR9) was performed by RT-PCR analysis. The expression patterns of PR9 showed that there is an accumulation of PR9 transcripts in leaves of FORL inoculated plants, 72h after inoculation (Figure 6). Furthermore, a noticeable accumulation of PR9 transcripts was detected in tomato leaves at 48 h and 72h after SA treatment (Figure 6).
|
Figure 6. PR9 transcript accumulation analyzed by RT-PCR in tomato leaves after 24h, 48h and 72h of 200µM SA roots feeding (lanes 1, 2, 3) compared to untreated plant (lane 4) and to infected plant by FORL 24h, 48h and 72h after inoculation (lane 5,6,7). Totub is the constitutive gene of Tubulin in tomato used as positive control. |
3.7. Accumulation of PR transcripts in tomato plants
The PR proteins are a group of inducible proteins whose synthesis is associated with some types of resistance to pathogen and elicitors. Thus, we study the expression of three different PR proteins. The electrophoresis profiles showed an accumulation of PR4 (glucanase), PR2 (chitinase) and PR1 (defensine) transcripts in leaves of plants inoculated by FORL only 72h after inoculation. In plants treated with 200µM SA a high level of PR4 and PR2 was detected earlier at 48h and 72h post treatment. However, very low PR1 gene expression was observed for the same treatment (Figure 7)
|
Figure 7. PR proteins transcripts accumulation analyzed by semi quantitative RT-PCR in tomato leaves after 24h, 48h and 72h of 200µM SA roots feeding (lanes 1, 2, 3) compared to untreated plant (lane 4) and to infected plant by FORL 24h,48h and 72h (lane 5,6,7). Totub is the constitutive gene of tubulin in tomato used as positive control. |
4. Discussion
Pre-treatment of plants with different biotic (pathogens and insect pests) and abiotic inducers (chemicals) induce plant defense against their subsequent attack. This induction of plant defense is mediated through various physiological, biochemical and molecular mechanisms (Idrees et al., 2011). In this study, we showed that SA can activate systemically the resistance against FORL in hydroponically grown tomato plants. This was accomplished by providing 200 μM of SA directly to the root system of the plant. The growth rates of FORL mycelium after the treatments with different concentrations of SA did not show any significant impact, which is on correlation with the result found by Juane (2010). It has been proposed that SA affects the plant growth under stress through nutrient uptake, water relations, stomatal regulation and photosynthesis (Hayet et al., 2009). SA treated plants showed healthy appearance and an optimal vegetative growth. These results indicate that SA has several beneficial effects including the enhancement of plant growth (Raskin, 1992) related to signaling pathways. In each experiment performed, there was a significant decrease in the incidence of disease when the pathogen inoculation was preceded by SA treatment. Plants that did not receive SA consistently had larger vascular browning and leaf yellowing than the treated plant.
Exogenous application of 200 µM SA on tomato plants through hydroponic medium could significantly increase foliar SA levels and activates systemic resistance that was effective against Alternaria solani (Spletzer et al., 1999). Exogenous SA stimulated the systemic resistance against Fusarium wilt of chickpea and reduced the disease severity significantly (Saikia et al., 2003). In this study it was observed that SA did not possess direct antifungal activity against F. oxysporum f. sp. radisiclycopersici, and disease resistance in tomato was the result of plant defense mechanisms rather than direct inhibitory effects of SA on the fungus. This strengthens the hypothesis that SA activates the signal transduction pathway, thus leading to the expression of SAR, rather than inhibiting the fungus directly (Métraux et al., 2002)
SA is known to be a potent inducer of systemic resistance in tobacco, cucumber, potato, and Arabidopsis (Coquoz et al., 1995; Ward et al., 1991; White et al., 1979). Consequently, it is not surprising that SA exerts a similar effect in tomato. This is in agreement with other reports showing that the treatments of plants with SA can provide a significant degree of protection against pathogens (Métraux et al., 1993; Kauss et al., 1993; Van Kan et al., 1995) as well as delaying the development of disease. Current findings revealed salicylic acid induced resistance to grey mould (Botrytis cinerea) on tomato and tobacco plants (Achuo et al., 2004).
In addition to delaying the development of disease, SA also affected the accumulation of phenolic compounds which are associated with plant resistance (El Hadrami et al., 1997). Phenolic compounds are naturally antifungal compounds and their accumulation in plants reduces pathogen attacks (Mpiga et al., 1997). In addition to direct effects of phenolic compounds on fungal pathogen, they are oxidized, by peroxidase, to form more toxic quinines (Gogoi et al., 2001). The study also showed that SA is an elicitor that stimulated the accumulation of the PAL transcripts in tomato leaves. This gene codes for an enzyme catalyzing biosynthetic reactions form defensive compounds (Sgarbi et al., 2003; Solecka and Kacperska, 2003; Zhao et al., 2005). It has been suggested that SA inhibits catalase activity, leading to increased levels of H2O2 (Chen et al., 1993), which in turn induces PAL gene expression (Desikanet al., 1998) and synthesis of phenolic compounds (Dorey et al., 1997). Evidence has shown that the PAL activity could be induced by SA elicitation in parsley, citrus and grapes (Chen et al., 2006; Lafuente et al., 2004; Thulke and Conrath, 1998), which would result in the accumulation of plant secondary metabolites (Janas et al., 2002; Sgarbi et al., 2003; Soleckaand Kacperska, 2003; Zhao et al., 2005). In addition, we found that in both SA-treated and infected untreated plants different phenolics of the hydroxycinnamic derivatives group were accumulated. These phenolics were different from those expressed by a healthy untreated plant. In summary, the results indicate that SA protects tomato plants from Fusarium wilt and could induces tomato resistance by enhancing induced chemical defenses.
Peroxidases are not only constitutive but also inducible enzymes, which are synthesized under the influence of various physical, chemical, and biotic agents (Haluskova et al., 2009). the peroxidase activity increased to a great extent in the SA treated plants compared to control, probably contributing in enhanced resistance of tomato to FORL. Spraying SA on pear plants increased peroxidase activity greatly and contributed in protection of pear fruits against postharvest diseases (Cao et al., 2006). A rapid increase in peroxidase activity was found also in pretreated infected faba bean plants after 24 h for B. fabae pathogen and after 48h for B. cinerea (Maggie et al., 2007). The role of oxidative enzymes such as peroxidase could be explained as an oxidation process of phenol compounds to oxidized products (quinones) which may limit the fungal growth. Vance et al. (1980) and Fry (1982) stated that peroxidase is known to be involved in the oxidation of polymerization of hydroxycinnamyl alcohols to yield lignin and crosslinking isodityrosine bridges in cell wall. Ride (1983) and Tarradet al. (1993), reported that increase in peroxidase activity, enhance lignification in response to chocolate spot infection which may restrict the fungal penetration. These findings indicate a positive relationship between resistance and peroxidase activity. Peroxidase also produces free radicals and hydrogen peroxide which are toxic to many microorganisms (Pena and Kuc, 1992). Another supportive suggestion was brought by Nawar and Kuti (2003) who stated that an increase in peroxidase activity is considered as a preliminary indicator for resistance of broad beans to chocolate spot disease. According to Igor (2014), this increase in peroxidase activity may be considered as defense function of the plant organism view the highest peroxidase activity observed in the calli growing on the medium containing SA and infected by bunt and smut pathogens. These compounds act as barriers against pathogen invasion. The study of gene expression encoding peroxidase (PR9) confirms the results found following the analysis of the peroxidase activity.
It is evident that SA is an endogenous signal for the activation of certain plant defense responses, including PR-gene expression and establishment of enhanced resistance (Klessig et al., 2000). In the present work the results obtained after 200 µM SA roots treatment of tomato plants revealed the over-expression of PR2 and PR4 genes in leaves at 48h and 72h post treatment. Exogenous application of SA induced expression of the same set of nine defense related-genes as were activated systemically after infection by TMV (Durner et al., 1997; Bidyut et al., 2013). Altogether, our results are supported by previous works indicating that the SA is involved in the regulation of induced immunity in tomato after induction of PRs protein expression locally and systemically in which SA effect induced the PR protein, chitinase, β-1, 3-glucanase and peroxidase enzyme activity, (Ryals et al., 1996; Wobbe and Klessig 1996; Durner et al., 1997; Bokshi et al., 2003). Finally, the results presented here show that exogenously applied SA induces a set of plant defense reactions affecting the plant pathogen invasion. Thus, SA could be a valuable alternative to disease control.
5. References
Achuo, E.A.,Audenaert, K.,Meziane, H., and Hofte, M. (2004). The salicylic acid dependent defense pathway is effective against different pathogens in tomato and tobacco. Plant Pathology, 53, 65-72.
Benhamou, N.and Bélanger, R. (1998). Benzothiadiazole-Mediated Induced Resistance to Fusariumoxysporum f. sp. radicis-lycopersiciin Tomato. Plant Physiol, 118, 1203-1212.
Benhamou, N., (1996). Elicitor-induced plant defence pathways. Trends Plant Sci, 1,233-240.
Benhamou, N., Lafontaine, P. J., and Nicole, M. (1994). Induction of systemic resistance to fausarium crown and root rot in tomato plants by seed treatment with chitosan. Phytopathology, 84, 1432-1444.
Bidyut, N., and Sinha B. S. P. (2013).Mechanism of action of salicylic acid during defense response of plant.IndianJ.Sci.Res, 4(1), 101-104.
Bokshi, A.I., Morris,S.C.,andDeverall, B.J. (2003). Effects of benzothiadiazole and acetylsalicylic acid on β-1, 3-glucanase activity and disease resistance in potato.Plant Pathology, 52, 22-27.
Cao, J., Zeng, K., and Jiang, W. (2006). Enhancement of postharvest disease resistance in Ya Li pear (Pyrusbretschneideri) fruit by salicylic acid sprays on the trees during fruit growth, Eur. J. Plant Pathol., 114, 363-370.
Chen, J.Y., Wen, P.F., Kong, W.F., Pan, Q.H., Zhan, J.C., Li, J.M., Wan, S.B., and Huang, W.D. (2006). Effect of salicylic acid on phenylpropanoids and phenylalanine ammonialyase in harvested grape berries. Postharvest Biol. Technol, 40, 64-72.
Chen Z., Silva,S., and Klessig, D. (1993). Active oxygen species in the induction of plant systemic acquired resistance by salicylic acid. Science, 262, 1883-1886.
Coquoz, J.L., Buchala, A. J., Meuwly, P., and Métraux, J.-P. (1995). Arachidonic acid induces local but not systemic synthesis of salicylic acid and confers systemic resistance in potato plants to Phytophthorainfestans and Alternariasolani. Phytopathology, 85, 1219-1224.
Daniel, O.J.A., Marleide, M.A. L., Luciane, V. R., and Márcia, V. S. (2008). Differential gene expression, induced by salicylic acid and f. sp. infection, in tomato. Pesq.agropec. Bras, Brasília, 431017-1023.
Dixon, R.A., Paiva, N.L., (1995). Stress-induced phenylpropanoids metabolism. Plant Cell, 7, 1085–1097.
Desikan, R., Reynolds, A., Hancock,J.T., and . Neill, S.J,(1998). Harpin and hydrogen peroxide both initiate programmed cell death but have differential effects on defense gene expression in Arabidopsis suspension cultures. Biochemistry Journal, 330, 115-120.
Dorey S., Baillieul, F., Pierrel, M.A., Saindrenan, P., Fritig, B., and Kauffmann, S.(1997). Spatial and temporal induction of cell death, defence genes, and accumulation of salicylic acid in tobacco leaves reacting hypersensitively to a fungal glycoprotein elicitor. Molecular Plant-Microbe Interactions, 11, 1102–1109.
Durner, J., Shah,J.,and Klessig, D.F.(1997). Salicylic acid and disease resistance inplants.Trends in Plant Science, 2, 266-274.
EL Hadrami, I., and d’Auzac, J. (1992). Effects of growth regulators on polyamine content and peroxidase activity in Heveabrasilensis callus. Ann. Bot, 69,323-325.
El Hadrami, I., Ramos, T., El Bellaj, M., El Idrissi-Tourane, A., and Macheix, J.J. (1997). A sinapic derivative as induced defense compound of date palm against Fusariumoxysporumf.sp.albedinis, the agent causing bayoud disease.J Phytopathology, 145,329-333.
Ernesto, P. B., Arjen,T.H., John, W.,van,T.K., Jan, A.L. and van, K. (1998). Fungal and plant gene expression during synchronized infection of tomato leaves by Botrytis cinerea. Eur. J. Plant Pathol., 104, 207–220.
Fry, S.C. (1982). Isodityrosine a new amino acid from plant cell wall glycoprotein. Biochem. J., 204, 449-455.
Gogoi, R., Singh, D. V., and Srivastava, K. D. (2001). Phenols as a biochemical basis of resistance in wheat against karnal bunt. Plant Pathology, 50, 470-476.
Haluskova, I., Valentovicova, K., Huttova, J., Mistrik, J., and Tamas, L. (2009). Effect of abiotic stresses on glutathione peroxidase and glutathione S-transferase activity in barley root tips.PlantPhysiol Biochem,47,1069-1074.
Hayat, Q., Hayat, S., Irfan, M.,and Ahmad, A. (2009). Effect of exogenous salicylic acid under changing environment: A review. Environ. Exp. Bot., 68,14-25.
Idrees, M., Naeem, N., Aftab, T., and Khan, M.M.A.(2011). Moinuddin. Salicylic acid mitigates salinity stress by improving antioxidant defense system and enhances vincristine and vinblastine alkaloids production in periwinkle [Catharanthus roseus (L.) G. Don]. Acta Physiol Plant,33,987-99.
Igor,M., Natalia,T., Olga,S. and Ekaterina, C. (2014). Salicylic acid increases the defense reaction against bunt and smut pathogens in wheat calli, Journal of Plant Interactions, 9, 306-314.
Janas, K.M., Cvikrová, M., Palagiewicz, A., Szafranska, K., and Posmyk, M.M.(2002). Constitutive elevated accumulation of phenylpropanoids in soybean roots at low temperature. Plant Sci, 163, 369-373.
Juane,D., Guowei, W., Zongsuo, L. (2010). Accumulation of salicylic acid-induced phenolic compounds and raised activities of secondary metabolic and antioxidative enzymes in Salvia miltiorrhiza cell culture.Journal of Biotechnology, 148, 99-104.
Kauss, H., Theisinger-Hinkel, E.,MindermannR., and Conrath, U. (1992). Dichloroisonicotinic and salycilic acid, inducers of systemic acquired resistance,enhance fungal elicitor responses in parsley.cells.Plant Journal, 2, 655-660.
Klessig, D.F., Durner, J., Noad, R., Navarre, D.A., Wendehenne, D., Kumar, D., Zhou, J.M., Shah,J.,Zhang, S., Kachroo, P., Trifa, Y., Pontier D., Lam E. and Silva, H. (2000). Nitric oxide and salicylic acid signaling in plant defense. Proc. Natl Acad. Sci., 8849-8855.
Lafuente, M.T., Sala, J.M., Zacarias, L. (2004). Active oxygen detoxifying enzymes and phenylalanine ammonia-lyase in the ethylene-induced chilling tolerance in citrus fruit.J.Agric. Food Chem. 52, 3606-3611.
Maggie, E.M.H.,Saieda, S., El-Abbasi,I.H.,andMikhail, M.S. (2007). Changes in Peroxidase Activity Due to Resistance Induced Against Faba Bean Chocolate Spot Disease. Egypt. J. Phytopathol, 35, 35-48.
Métraux, J.P., Nawrath, C., and Genoud T. (2002). Systemic acquired resistance.Euphytica, 124, 237-243.
Métraux, J.P., and Raskin, I.(1993). Role of phenolics in plant disease resistance. In: Biotechnology in plant Disease Control. New York: Wiley-Liss Inc.
Mpiga, P., Belange, R. R., Paulitz, T. C., and Benhamou, N. (1997). Increased resistance to Fusariumoxysporumf.sp. radis– lycopersici in tomato plants treated with the endophytic bacterium Pseudomonas fluorescens strain 63-28. Physiological and Molecular Plant Pathology, 50, 301-320.
Nawar, H.F., and Kuti, J.D. (2003). Wyerone acid phytoalexin synthesis and peroxidase activity as markers for resistance of broad beans to chocolate spot disease.J.Phytopathol, 151, 564-570.
Pena, M., and Kuc, J.A. (1992). Peroxidase-generated hydrogen peroxidase as a source of antifungal activity in vitro and on tobacco leaf disks.Phytopathology, 82,696-699.
Ragiba, M., Vamsi, J. N., Hyeonju L., Harold, N. T., Yanhong, D., and Jyoti, S. (2012). Salicylic Acid Regulates Basal Resistance to Fusarium Head Blight in Wheat. The American Phytopathological Society, 25, 431-439.
Raskin, I. (1992). Role of salicylic acid in plants. Ann.Rev. of Plant Pysiol.and PlantMol. Biol., 43, 439-463.
Ride, J.P. (1983). Cell walls and other structural barriers in defence. In: J.A. Calloz (ed.),Biochemical Plant Pathology., . New York, USA: John Wiley and Sons.
Ryals, J.A., Neunschwander, U.H., Willits, M.G., Molina, A.,Steiner, H.Y., and Hunt,M.D. (1996). Systemic acquired resistance. Plant Cell, 8, 1809-1819.
Saikia, S., Singh, T., Kumar, R., Srivastava, J., Srivastava, A.K., Singh, K., and Arora, D.K. (2003). Role of salicylic acid in systemic resistance induced by Pseudomonas fluorescens against Fusarium oxysporum f. sp. ciceri in chickpea. Microbiol. Res., 158, 203-213.
Sgarbi, E., Fornasiero, R.B., Lins, A.P., and Bonatti, P.M. (2003). Phenol metabolism is differentially affected by ozone in two cell lines from grape (Vitis vinifera L.) leaf. Plant Sci., 165, 951-957.
Sharma, H.C., Sujana, G, and Rao, DM (2009). Morphological and chemical components of resistance to pod borer, Helicoverpaarmigera in wild relatives of pigeonpea.Arthropod.Plant Interactions, 3, 151-161.
Solecka, D., and Kacperska, A. (2003). Phenylpropanoid deficiency affects the course of plant acclimation to cold. Physiol. Plantarum, 119, 253-262.
Song, W., Zhou, L., Yang, C., Cao, X., Zhang, L., and Liu, X. (2004). Tomato Fusariumwilt and its chemical control strategies in a hydroponic system.Crop Protection, 23,243-247.
Spletzer, M.E. Enyedi, A.J. (1999). Salicylic acid induces resistance to Alternariasolani in hydroponically grown tomato. Phytopathology, 89, 722-727.
Sudhamony, M., Nirupama, M., and Adinpunya, M. (2009). Salicilic acid-induced resistance to Fusariumoxysporum f. sp. Lycopersiciintomato.Plant Physiology and Biochemistry, 47, 642-649.
Tarred, A.M., El-Hyatemy, Y.Y.,andOmar, S.A. (1993). Wyerone derivatives and activities of peroxidase and polyphenol oxidase in faba bean leaves as induced by chocolate spot disease. Plant Sci., 89, 161-165.
Thulke, O., and Conrath, U., (1998). Salicylic acid has a dual role in the activation of defenserelated genes in parsley. Plant J., 14, 35-42.
Vakalounakis, D.J. and Fragkiadakis G.A. (1999). Genetic diversity of Fusariumoxysporumisolates from cucumber: differentiation by pathogenicity,vegetative compatibility and RAPD fingerprinting. Phytopathology, 89,161-168.
Vance, C.P., Kirk, T.K.,andSherwood, R.T. (1980). Lignification as a mechanism of disease resistance.Annu. Rev. Phytopathol., 18, 259-288.
Van Kan, J.A.L., Cozijnsen, T., Danhash,N., and . De Wit, P.J.G.M. (1995). Induction of tomato stress protein mRNAs by ethephon, 2, 6-dichloroisonicotinic acid and salycilate.Plant Molecular Biology, 27, 1205-1213.
Walters, D., Walsh, D., Newton, A., and Lyon, G. (2005). Induced resistance for plant disease control: Maximizing the efficacy of resistance elicitors. Phytopathology 95, 1368-1373.
War, A.R., Paulraj, M.G., War, M.Y., and Ignacimuthu S. (2011).Jasmonic acid-mediated induced resistance in groundnut (Arachishypogaea L.) against Helicoverpaarmigera (Hubner) (Lepidoptera: Noctuidae). J Plant Growth Regul; 30, 512-523
Ward, E. R., Uknes, S. J., Williams, S. C., Dincher, S. S., Wiederhold, D.L., Alexander, D. C., Ahl-Goy, P., Métraux, J.-P., and Ryals, J. A. (1991).Coordinate gene activity in response to agents that induce systemic acquired resistance. Plant Cell, 3, 1085-1094.
White, R. F. (1979). Acetylsalicylic acid (aspirin) induces resistance to tobacco mosaic virus in tobacco. Virology, 99, 410-412.
Wobbe, K.K.,and Klessig, D.F. 1996. Salicylic acid: an important signal in plants. In DPS Verma (Ed), Plant Gene Research: Signal Transduction and Development (pp. 167 196).Wien, Austria.
Yukimune, Y., Tabata, H., Higashi, Y., and Hara, Y. (1996).Methyl jasmonate-induced overproduction of paclitaxel and baccatin III in Taxus cell suspension cultures.Nat.Biotechnol. 14, 1129–1132.
Zhao, J., Davis, L.T., and Verpoort, R. (2005). Elicitor signal transduction leading to production of plant secondary metabolites. Biotechnol.Adv, 23, 283–333.Zhang, C., Yan, Q., Cheuk, W., and Wu, J. (2004). Enhancement of tanshinone production in Salvia miltiorrhiza hairy root culture by Ag+ elicitation and nutrient feeding. Planta Med, 70, 147-151.