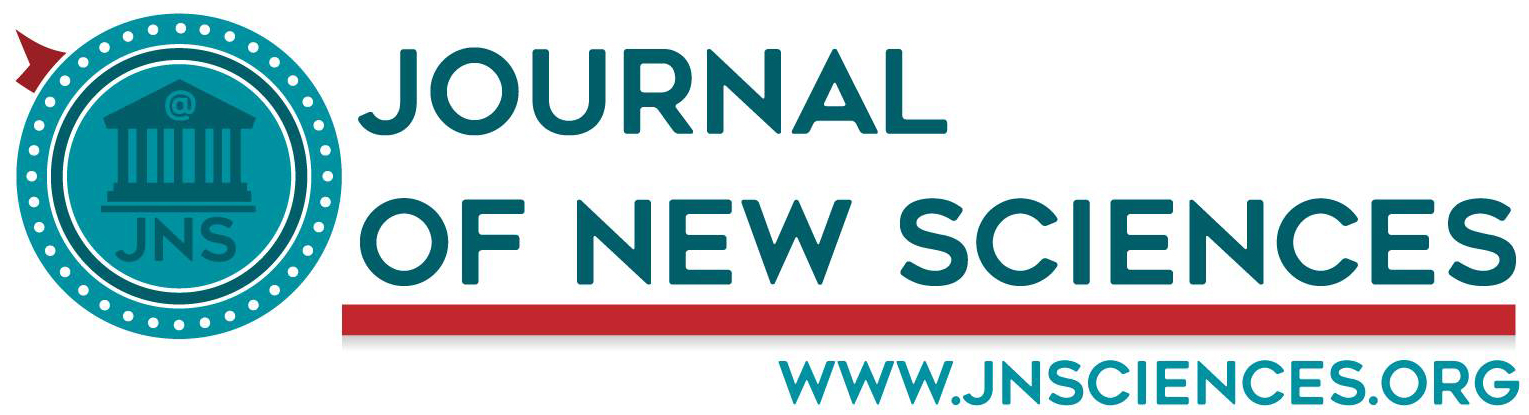
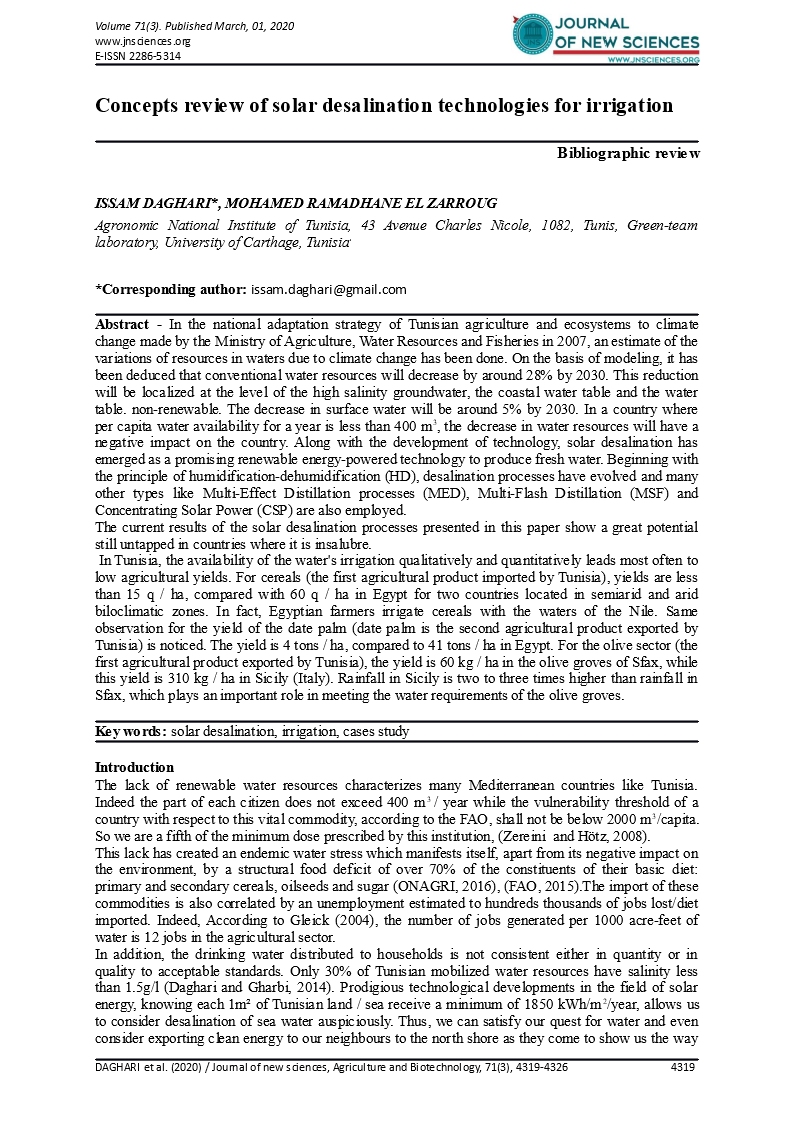
- Category: Volume 71
- Hits: 5478
Concepts review of solar desalination technologies for irrigation
Bibliographic review
ISSAM DAGHARI
MOHAMED RAMADHANE EL ZARROUG
Agronomic National Institute of Tunisia, 43 Avenue Charles Nicole, 1082, Tunis, Green-team laboratory, University of Carthage, Tunisia,
Abstract - In the national adaptation strategy of Tunisian agriculture and ecosystems to climate change made by the Ministry of Agriculture, Water Resources and Fisheries in 2007, an estimate of the variations of resources in waters due to climate change has been done. On the basis of modeling, it has been deduced that conventional water resources will decrease by around 28% by 2030. This reduction will be localized at the level of the high salinity groundwater, the coastal water table and the water table. non-renewable. The decrease in surface water will be around 5% by 2030. In a country where per capita water availability for a year is less than 400 m3, the decrease in water resources will have a negative impact on the country. Along with the development of technology, solar desalination has emerged as a promising renewable energy-powered technology to produce fresh water. Beginning with the principle of humidification-dehumidification (HD), desalination processes have evolved and many other types like Multi-Effect Distillation processes (MED), Multi-Flash Distillation (MSF) and Concentrating Solar Power (CSP) are also employed. The current results of the solar desalination processes presented in this paper show a great potential still untapped in countries where it is insalubre. In Tunisia, the availability of the water's irrigation qualitatively and quantitatively leads most often to low agricultural yields. For cereals (the first agricultural product imported by Tunisia), yields are less than 15 q / ha, compared with 60 q / ha in Egypt for two countries located in semiarid and arid biloclimatic zones. In fact, Egyptian farmers irrigate cereals with the waters of the Nile. Same observation for the yield of the date palm (date palm is the second agricultural product exported by Tunisia) is noticed. The yield is 4 tons / ha, compared to 41 tons / ha in Egypt. For the olive sector (the first agricultural product exported by Tunisia), the yield is 60 kg / ha in the olive groves of Sfax, while this yield is 310 kg / ha in Sicily (Italy). Rainfall in Sicily is two to three times higher than rainfall in Sfax, which plays an important role in meeting the water requirements of the olive groves.
Key words: solar desalination, irrigation, cases study
Introduction
|
The lack of renewable water resources characterizes many Mediterranean countries like Tunisia. Indeed the part of each citizen does not exceed 400 m3 / year while the vulnerability threshold of a country with respect to this vital commodity, according to the FAO, shall not be below 2000 m3/capita. So we are a fifth of the minimum dose prescribed by this institution, (Zereini and Hötz, 2008). This lack has created an endemic water stress which manifests itself, apart from its negative impact on the environment, by a structural food deficit of over 70% of the constituents of their basic diet: primary and secondary cereals, oilseeds and sugar (ONAGRI, 2016), (FAO, 2015).The import of these commodities is also correlated by an unemployment estimated to hundreds thousands of jobs lost/diet imported. Indeed, According to Gleick (2004), the number of jobs generated per 1000 acre-feet of water is 12 jobs in the agricultural sector. In addition, the drinking water distributed to households is not consistent either in quantity or in quality to acceptable standards. Only 30% of Tunisian mobilized water resources have salinity less than 1.5g/l (Daghari and Gharbi, 2014). Prodigious technological developments in the field of solar energy, knowing each 1m² of Tunisian land / sea receive a minimum of 1850 kWh/m2/year, allows us to consider desalination of sea water auspiciously. Thus, we can satisfy our quest for water and even consider exporting clean energy to our neighbours to the north shore as they come to show us the way by the megaproject TuNurthatwill erect solar power tower 2 GW from 100 km² of Tunisian land. The World Bank study (DLR, 2007) analyzes the potential of solar thermal energy technology for large-scale desalination of seawater in the Middle East and North Africa (MENA). It provides a comprehensive database of technology options. The study provides a first information base for a policy framework. It quantifies solar energy resources and the expected cost of the desalinated water. In this study, the potential for thermal desalination of seawater can reach 85 billion cubic meters of water, which is very good for Tunisia. Desalination will be a serious option to fight against the impacts of climate change in Tunisia. In the following paragraphs, a literature review on the subject has been made. |
I -Solar energy experiments in desalination for irrigation
Frank Shuman, built a solar thermal power station in Maadi, Egypt (Ragheb, 2011). Shuman’s plant used parabolic troughs which convert heat into electricity through a converter to power a 60-70 horsepower engine that pumped a little more than 22 cubic meter of water per minute from the Nile River to irrigate the adjacent cotton fields (Fig.1) but over the First World War, the facility was abandoned.
|
Figure 1: Concentrated solar power plant in Maadi (Egypt), built in1897(RAGHEB, 2011) |
Thereafter, the process humidification/dehumidification (HD) was used. In this desalination process, the moist air is used as a mean transporting the vapor. The first experiments have shown that researchers have integrated solar panels to greenhouse, so the idea to combine a solar still with a greenhouse is not newest. Previously, Trombe and Foex (1961) described similar system and experiences suchlike have gained momentum. In 1979 at the University of Hanover in Germany, a closed greenhouse was built. This desalination system, called the ITG-system, was based on evaporated water by solar energy and condensed water on the enclosure (Fig.2).
The simplicity of the process imitates the hydrological cycle where seawater heated by the sun evaporates, cools down to form clouds, and returns to the earth as rain, fog or dew.
Working in the same thematic, in the period 1978 to 1981, Dumont and De Cachard (1984) proposed in their study a greenhouse with solar distillation using a developed Bettaque concept (Chouaieb, 2007). According to Chaibi (2000), the results obtained from the experiments since 1978 show that the average daily production of fresh water has been in the range of 2-3.5 l/m2/day under the most favourable conditions.
A comparison between modified Bettaque system and ITG-system shows the increase of the ITG-system efficiency compared to Bettaque system, 29% against 16%, and a productivity of 2-2.5 l/m2/day in the ITG-system against 1 1/m2/day. As a conclusion, that this system is more adapted to arid regions.
|
Figure 2: Integrated greenhouse, ITG-system (STRAUCH, 1985), (CHAIBI, 2000) |
So, these are small experiments that have been made many decades ago but in the last twenty years, things took more wingspan. The first big pilot project suchlike was launched in 1992 in the Canary Island of Tenerife known as the ‘Garden of the Gods’, but now it was abandoned and seriously damaged by excessive extraction of ground water(Paton and Davies, 1996). The results from this pilot project validated the concept and demonstrated the potential for other arid regions.
Since the Tenerife greenhouse, two further research centers done by the same project on ‘Seawater Greenhouse Development’ were established in order to test the technology in more extreme climates, increase water production rates, research crop yields and improve climate control. The second Seawater Greenhouse was constructed on Al-Aryam Island, Abu Dhabi, United Arab Emirates in 2000.Crop production in terms of quality and quantity has been outstanding. The third system and joint research facility was completed in 2004 near Muscat, Oman in collaboration with Sultan Qaboos University, (Davies and Paton, 2005).
In this last project, thermodynamic and economic efficiencies of solar desalination systems were determined for various greenhouse related-parameters on desalination process. Simulation studies have shown that the dimensions of the greenhouse had the greatest overall effect on the water production and energy consumption. Low power consumption went hand-in-hand with high efficiency. A wide shallow greenhouse, 200 m wide by 50 m deep gave 125m3 per day of fresh water. This was greater than a factor of two compared to the worst-case scenario with the same overall area (50 m wide by 200 m deep), which gave 58 m3 per day. Low power consumption went hand in hand with high efficiency (Fig.3). The wide shallow greenhouse consumed 1.16 kwh/m3, while the narrow deep structure consumed 5.02 kwh/m3. Total fresh water production for three different versions (temperate, tropical and oasis) was also calculated,(MAHMOUDI et al., 2010). The model results predict that the Seawater Greenhouse will perform efficiently throughout the year, but with measurable variations in performance between the alternative versions.
|
Figure3: Seawater GreenhouseinSultanQaboos University, Oman (MAHMOUDIet al., 2010) |
The results of this pilot plant operation clearly indicated that desalinated water produced by the greenhouse was much less than the design values and it was due to leakages and non-availability of low temperature cooling water. The solution is to reduce the temperature of the condenser and make it even colder. An alternative could be the use of deep seawater (lower temperatures) to improve the performance of the condenser.
Furthermore, a new technology has emerged since the 2000s called Condensation Irrigation (CI) based on humidification dehumidification process but a slightly different from HD process used in Sultan Qaboos University. In this case, the humidified air is condensed in the ground and not by using a condenser. In fact, water passes through solar panels and then is pumped into a storage tank. The heated water moistens the ambient air thereby the formed vapor becomes a humidified air which is ventilated into an underground pipe system. This fresh water will precipitate as the air is cooled by the ground. By using drainage pipes for underground air transportation, perforations in the pipes enable the water to percolate into the soil.
The CI system has attracted attention from several North African countries, and pilot plants are now in operation in Tunisia and Algeria (Fig. 4). In Tunisia, for example, with favourable weather conditions, the results showed that the production of fresh water daily calculated is 1.8 kg m -2. Condensate was sufficient for 30% of the water requirement of a mature tomato crop (Chouaib and Chaibi, 2014).
|
Figure 4: shows a diagram of the experimental system. This unit includes:,(A, C, D) solar panels and a fan, inducing the processes of air humidification (evaporation) and dehumidification of the air (condensation), a storage tank (B) (Chouaieb, 2007). |
II -The use of solar desalination for irrigation: cases study
Solar desalination technology has evolved from simple HD process to others processes more advanced such as Multi-Effect Distillation processes (MED), Multi-Flash Distillation (MSF) and Concentrating Solar Power (CSP), although the HD process is still used but developed as the case system in Qatar detailed in this paragraph.
Several companies have hatched in the world in the field of solar desalination technologies for irrigation. For example, Sun drop Farms which is an Australian leader in sustainable horticulture for the arid world are using seawater and sunlight for growing high-value crops, (KAYE, 2014).
It’s an agri business company with the technology of know-how to develop and operate greenhouses in locations that have little or no access to arable land, fresh water sources, or grid energy. They have created a proprietary food production system which grows high-quality produce year-round in greenhouses that use the abundant and renewable resources of sunlight and seawater.
As of 2015, Sun drop Farms is constructing a 20 ha solar-powered greenhouse facility near its original site, south of Port Augusta in South Australia. Sun drop Farms operations will be primarily powered by a new concentrated solar thermal power plant and seawater will be withdrawn from Spencer Gulf and desalinated to feed produce. 11,000 parabolic mirrors will redirect sunlight on a 115 meter tall tower, which in turn will create steam that will power turbines and then provide energy for the mega-greenhouses. The project also promises 200 jobs and will produce over 15,000 tonnes of tomatoes a year for metropolitan markets across Australia (THE GUARDIAN, 2012). A $150 million development contract was awarded in 2014 to construct the expanded facility over an 18-24 month time frame and the total project cost is an estimated $205 million.
A similar solar thermal project was launched in 2014 in another water-stressed region. Water FX, a California start-up, has built a solar thermal-powered desalination plant in the San Joaquin Valley that cleans saline drainage water and turns into fresh water for irrigation. The plant will ultimately generate up to 3.8 billion liters, of clean water per year — enough water for 10,000 homes or 800 hectares of cropland. It will be built on 14hectares of lands currently used to grow salt-tolerant crops, and will recycle unusable irrigation water from a 2800 hectares drainage area into a new and much-needed source of freshwater for nearby water districts by removing unwanted mineral and salts(PHILIPS,2014).
The market for desalination components will top $5 billion this year, according to forecasts from experts on the topic, with drought-stricken California is at the epicenter of desalination activity (Hardcastle, 2015). They will buy the solar desalinated water by the acre-foot and then sell it on to California farmers. Water FX total operating costs are approximately $450 for1.2 million liters, (KELLEY-DETWILER, 2014), it is an extraordinary price and project officials say that investissements of costs may decline further.
Another project in the same sens, a pilot facility in Qatar was built in 2012 under the Norwegian Sahra Forest Project which unveiled as a concept at the Copenhagen conference in 2009.Its originators was the UK architect Michael Pawlyn, who was involved in the “Eden Project”, the world biggest greenhouse, cucumbers were grown in a greenhouse cooled by seawater and irrigated by water desalinated by a mechanism powered by generation from concentrated solar power (CSP) .The electricity to drive various machines and facilities came from solar PV, (PARKINSON, 2012).
The heat from the Concentrated Solar Power mirrors is used to drive a multistage evaporative desalination system for production of distilled water for the plants in the greenhouse and outside. The waste heat is used to warm the greenhouses in the winter and to regenerate the desiccant used for dehumidifying the air.It’s the humidification-dehumidification (HD) processus but more developed using CSP. The estimated cost for the Pilot Plant is $ 5.3 millionover an area of 1 hectare, (Fig .5). The results were better than expected, (SCOTT, 2012).
|
Figure: 5 A saltwater-cooled greenhouse at the Sahara Forest Project's pilot facility in Qatar (HEIN, 2012) |
The Safari Forest Project says the next stage will be to move to a larger, 20ha facility, where the idea can be tested at a larger scale. The ultimate goal is to build truly large scale plants that could cover 4,000 hectares. This would include a solar thermal array of around 100MW, 300 hectares of saltwater greenhouses, 2,000 hectares of outdoor revegetation and cereal crops, and 150 hectares of algae cultivation.
According to experts of the Sahara Forest Project, the implementation of such mega-facility over 4 000 hectares, situated in the northern part of Africa, could as an example provide:
• Concentrating Solar Power (CSP) can power all the Oasis’ needs and exporting 325 GWh/ year
• Self-sustained thermal desalination of freshwater for all irrigation needs
• 300 hectares of saltwater greenhouses, yielding 190 000 annual tons of tomatoes and melons
• 2 000 hectares of new outdoor revegetation and crops, yielding 30 000 tons/year of fodder crops
• 150 hectares of algae cultivation, capable of yielding 7 500 tons/year of biofuel-ready algae oils
While the above is but one example of many possible scenarios, it illustrates how a major Sahara Forest Project scheme can yield substantial amounts of energy, food, fuel and fodder crops. This particular example could employ up to 20 000 people directly and support five times as many.
On 2014, the same firm signed an agreement for the creation of a similar project. The Center will be located near Aqaba, Jordan. The project could cover more than 3,000 hectares, employ more than 8,000 people and produce more than 500 GWh of electricity and 200,000 tonnes of fruits and vegetables each year. It is certain that if these projects succeed, we will see these agribusiness companies flourish.
Actually, Global Solar Energy Market to climb 36% in 2015 amid falling solar prices. New solar photovoltaic (PV) installations will reach 55,000 megawatts in 2015 - the highest annual total to date, researchers say. By 2020, global PV installations could jump to 135,000 megawatts for the year, and researchers said - enough to power the equivalent of roughly 20 million average U.S. homes (GALLUCI, 2015).
Annual investment on CSP Technology will jump from 21 billion euros to 174 billon euros between 2015 and 2050. Cumulative capacity evolves from 420 megawatts in 2015 to 1, 500,000megawatts in 2050, (JHA, 2009).
The electrical power requirements for the pumping of water will also be provided by solar PV, the price - without subsidies -is become very competitive. Indeed, as a concrete example, on 2014, following a tender launched last August, Brazil has signed a contract to take delivery, for 20 years from 2017, of electricity from 31 PV power plants with a total capacity of 1048 MWc at a price of $ 89 per MWh.
In Germany, German solar power plants produced a world record 22 Gigawatts of electricity per hour, equal to nuclear power stations at full capacity in Tunisia; we are not even to 55 MWc to date despite our 1850 kWh / year / m² of solar irradiance! The world is more than 200 GW.
According to MHIRI (2014), a 1.2 ha farm planted with olive trees in southern Tunisia irrigated by reverse osmosis desalinated water using electrical energy saw its gross margin evolving from 166 DT / ha under rainfed conditions to 7761 DT / ha, 11221 DT / ha and 11075 DT / ha respectively for the years 2011/2012, 2012/2013 and 2013/2014 following irrigation with desalinated water (1US $= 2.85 DT based on the 2019 exchange rate). The average desalinated water cost covering all expenses was 1.23 DT / m3 (MHIRI, 2018).
Another reverse osmosis desalination project was realized in the Gounat area in Mahdia gouvernorate in Tunisia to irrigate 3.5 ha (75 green houses with vegetable cops) during 2016. The desalinated water obtained has a salinity of 0.2 g/l whereas the aquifer salinity was 4.8 g/l. The blended water salinity was 1.5 g/l.
The additional energy costs generated by the electromechanical equipment of the desalination plant induces a total cost, including the pumping from drilling and the discharge to the basin of the mixture, of the order of 0.12 US$ per m3 without taking into account the investment cost of 405 thousand dinars excluding taxes. The network water price selled by the state is about 0.05 US$ per m3, (Dhahbi, 2016).
Conclusion
Solar desalination has evolved in recent years. The technology is well mastered. Desalination costs have dropped from $ 1US to $ 0.5 US / m3of desalinated water and even less in recent years. The newly solar approach must be carried out slowly but preliminary measures such as wáter saving and a wáter governance must be undertaken.
References
Davies, P. A.; Paton C. (2005)The seawater greenhouse in The United Arab Emirates: Thermal modelling and evaluation of design. Desalination options v.173 (2), p. 103-111. 2005.doi: 10.1016/j.desal.2004.06.211.
Chaibi, M.T. (2000)An overview of solar desalination for domestic and agriculture water needs in remote arid areas, Desalination, v. 127 (2), p.119-133. Available from: <https://doi.org/10.1016/S0011-9164(99)00197-6>.
Chaibi, M.T. (2013) Thermal Solar Desalination Technologies for Small-Scale Irrigation. American Journal of EnergyResearch, 1(2), 25-32.
Chouaieb W., (2007) Etude du systeme d’irrigation par evapo-condensation et sa contribution à la satisfaction des besoins en eaux de laculture de tomate sous serre. Thesis.
Chouaib, W.; Chaibi, M.T. (2014) Performance evaluation on condensation-irrigation solar system under arid climate conditions. Int. J. Energy Technology and Policy, v. 10, No. 2. Conference: IREC 2012. Available from: <https://www.researchgate.net/publication/272295737>.doi: 10.1504/IJETP.2014.066330.
Daghari I and Gharbi A.,(2014)Modelisation by SALTMOD of Leaching Fraction and Crops Rotation as Relevant Tools for Salinity Management in the Irrigated area of DyiarAlHujjej,Tunisia. International Journal of Computer and Information Technology (ISSN: 2279 – 0764) Volume 03 – Issue 04, July 2014.
Dhahbi, G.(2016) Final reportf the project Adaptation to Climate Change through Improved Water Demand Management in Irrigated Agriculture by Introduction of New Technologies and Best Agricultural Practice. Gneral Directorate of Agricultural Engeneering and Water Exploitation, Tunisia, p. 75.
DLR, (2007) Concentrating Solar Power for Seawater Desalination. AQUA-CSP Study Report.
Dument, M. ; DE Cachard, M. (1984).Plasticulture; 61- 11.Available from: <www.sciencedirect.com/science/article/pii/S>.
FAO.AQUASTAT.(2015) Availablefrom: <http://www.fao.org/nr/water/aquastat/data/cf/readPdf.html?f=TUN-CF_fra.pdf >.
Gallucci M., (2015) International Business Times. Global Solar Energy Market To Climb 36% In 2015 Amid Falling Solar Prices, Climate Change Concerns: Report. Available from: https://www.ibtimes.com/global-solar-energy-market-climb-36-2015-amid-falling-solar-prices-climate-change-1971207.
Hardcastle J.L., (2015) Environmental Leader. California’s First Commercial Solar Desalination Plant in the Works. Available from: https://www.environmentalleader.com/2015/07/californias-first-commercial-solar-desalination-plant-in-the-works/.
Hein T., (2012) Greenhouses Canada. Sahara Forest Project' includes seawater-based greenhouses. Available from: https://www.greenhousecanada.com/energy/efficiency/sahara-forest-project-includes-seawater-based-greenhouses-20154.
JHA A., (2009) Concentrated solar power could generate 'quarter of world's energy'.Available from: <https://www.theguardian.com/environment/2009/may/26/solarpower-renewableenergy>.
Mahmoudi, H.; Spahis, M.F.;Goosen, A.; Ghaffour, N.; Drouiche, N.andOuagued,A.(2010)Application of geothermal energy for heating and fresh water production in a greenhouse desalination unit: a case study from Algeria. Renew Sustain Energy Revue, v.14, p.512–7.Available from: <https://www.mobt3ath.com/uplode/book/book-4090.pdf>.
Kaye L. (2014)Can Solar Thermal Desalination Make Sustainable Agriculture Possible?,cleantechnical. Available from: <https://cleantechnica.com/2014/12/05/can-solar-thermal-desalination-make-sustainable-agriculture-possible/>. MHIRI, A. (2014)Final report on solar desalination extension site in the SASS bazin in Algeria, Morooco, Libya and Tunisia (in frensh) OSS. ISBN : 786973-79-1.2014.
Mhiri,A. (2018)L’agriculture tunisienne à la croisée des chemins, quelle vision pour une agriculture durable, ISBN : 978-9938-00-916-3 :Simpact imprimerie and edition, Tunis, 280p.2018. Parkinson, G., (2012)Solar and seawater turn desert into a greenhouse. RenewEconomy. Available from: <https://reneweconomy.com.au/solar-and-seawater-turn-desert-into-a-greenhouse-28833/>. Paton, C.; A. Davies. (1996)The seawater greenhouse for arid lands. Proc Mediterranean Conference on Renewables Energy Sources for Water Production,10-12 June 1996, Santorini, Greece.Availablefrom: <https://pdfs.semanticscholar.org/8759/3b5c88015464e5329ec347699f0b2313b454.pdf>. Philips,A. (2014)Have You Heard Of Solar Desalination? If Not, You Will Soon. Climate Progress.2014. Available from: <https://thinkprogress.org/have-you-heard-of-solar-desalination-if-not-you-will-soon-aa6dee9fb2ab/>. |
Kelly-DetwilerP., (2014)WaterFX Sees Solar Desalination As One Way To Address The World's Water Problem. Forbes/Energy. Available from: <https://www.forbes.com/sites/peterdetwiler/2014/01/07/waterfx-sees-solar-desalination-as-one-way-to-address-the-worlds-water-problem/>.
ONAGRI, 2016. Internal reports, Tunisia.Available from: <https://www.onagri.nat.tn -2016>.
Ragheb, M. (2011)Solar thermal power and energy storage historical perspective. University of Illinois at Urbana-Champaifn; 2011.Available from: <http://www.solarthermalworld.org/node/3303>.
THE GUARDIAN, (2012/nov/24) Growing food in the desert crisis.
Available from: <https://www.theguardian.com/environment/2012/nov/24/growing-food-in-the-desert-crisis>.
Trombe, F.;and Foex, M. (1961)Utilisation of solar still energy for simultaneous distillation of brackish water and air conditioning of hot houses in arid regions.In: UN Conference on new sources of energy, page paper 35/S/64, Rome. 11p.1961. Available from: <https://iwaponline.com/ws/article/18/5/1505/39273/Desalination-for-agriculture-water-quality-and>.
Scott, T., (2017)Improving Health and Well-Being in the Built Environment. Biophilicdesign.Sahara Forest Project, 2017, Qatar.Available from: <https://www.terrapinbrightgreen.com/.../Tapping-into-Nature-2017>.
Zereini, F.;Hötz,H., (2008) Climatic Changes and Water Resources in the Middle East and North Africa. eBook ISBN978-3-540-85047-2.4 ed. Springer-Verlag Berlin Heidelberg,2008. 552p.