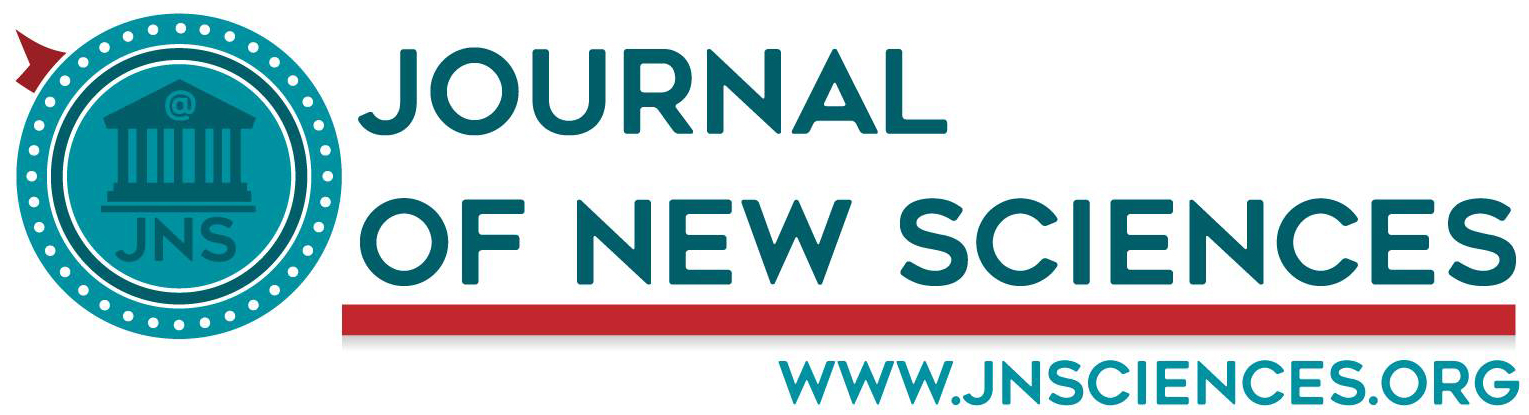
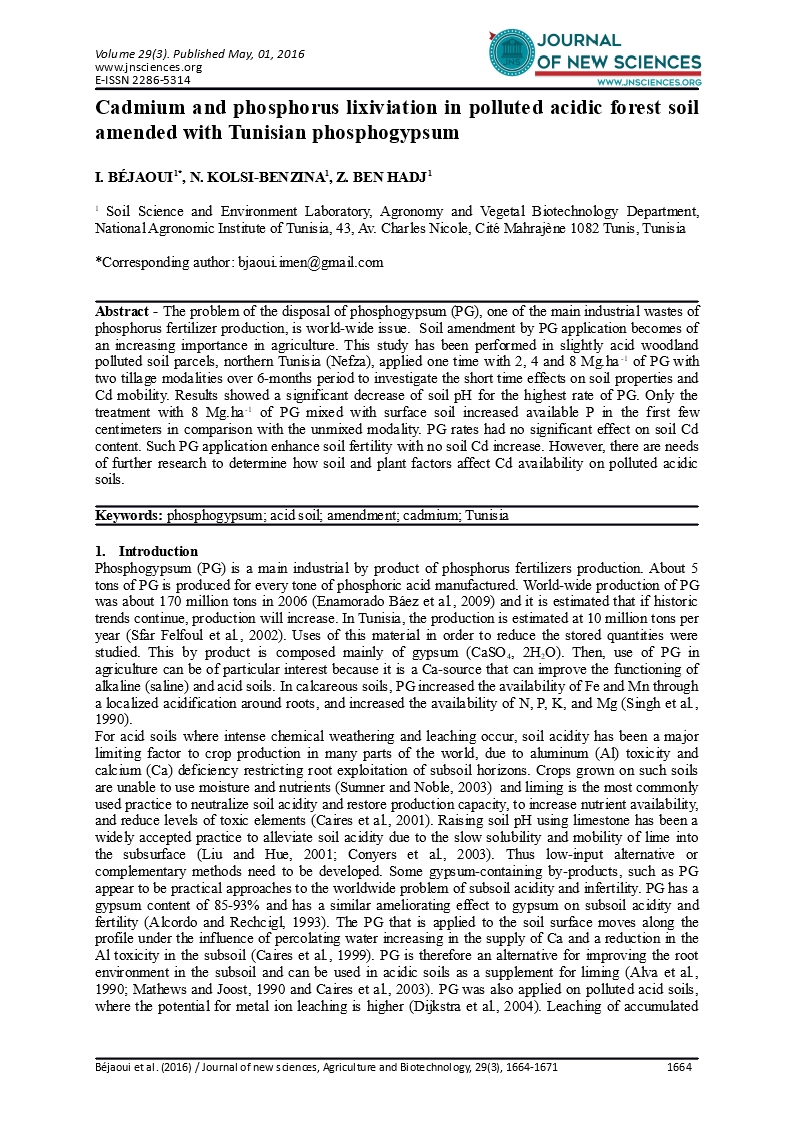
- Category: Volume 29
- Hits: 7658
Cadmium and phosphorus lixiviation in polluted acidic forest soil amended with Tunisian phosphogypsum
I. Béjaoui1*
N. Kolsi-Benzina1
Z. Ben Hadj1
1 Soil Science and Environment Laboratory, Agronomy and Vegetal Biotechnology Department, National Agronomic Institute of Tunisia, 43, Av. Charles Nicole, Cité Mahrajène 1082 Tunis, Tunisia
Abstract - The problem of the disposal of phosphogypsum (PG), one of the main industrial wastes of phosphorus fertilizer production, is world-wide issue. Soil amendment by PG application becomes of an increasing importance in agriculture. This study has been performed in slightly acid woodland polluted soil parcels, northern Tunisia (Nefza), applied one time with 2, 4 and 8 Mg.ha-1 of PG with two tillage modalities over 6-months period to investigate the short time effects on soil properties and Cd mobility. Results showed a significant decrease of soil pH for the highest rate of PG. Only the treatment with 8 Mg.ha-1 of PG mixed with surface soil increased available P in the first few centimeters in comparison with the unmixed modality. PG rates had no significant effect on soil Cd content. Such PG application enhance soil fertility with no soil Cd increase. However, there are needs of further research to determine how soil and plant factors affect Cd availability on polluted acidic soils.
Keywords: phosphogypsum; acid soil; amendment; cadmium; Tunisia
-
Introduction
Phosphogypsum (PG) is a main industrial by product of phosphorus fertilizers production. About 5 tons of PG is produced for every tone of phosphoric acid manufactured. World-wide production of PG was about 170 million tons in 2006 (Enamorado Báez et al., 2009) and it is estimated that if historic trends continue, production will increase. In Tunisia, the production is estimated at 10 million tons per year (Sfar Felfoul et al., 2002). Uses of this material in order to reduce the stored quantities were studied. This by product is composed mainly of gypsum (CaSO4, 2H2O). Then, use of PG in agriculture can be of particular interest because it is a Ca-source that can improve the functioning of alkaline (saline) and acid soils. In calcareous soils, PG increased the availability of Fe and Mn through a localized acidification around roots, and increased the availability of N, P, K, and Mg (Singh et al., 1990).
For acid soils where intense chemical weathering and leaching occur, soil acidity has been a major limiting factor to crop production in many parts of the world, due to aluminum (Al) toxicity and calcium (Ca) deficiency restricting root exploitation of subsoil horizons. Crops grown on such soils are unable to use moisture and nutrients (Sumner and Noble, 2003) and liming is the most commonly used practice to neutralize soil acidity and restore production capacity, to increase nutrient availability, and reduce levels of toxic elements (Caires et al., 2001). Raising soil pH using limestone has been a widely accepted practice to alleviate soil acidity due to the slow solubility and mobility of lime into the subsurface (Liu and Hue, 2001; Conyers et al., 2003). Thus low-input alternative or complementary methods need to be developed. Some gypsum-containing by-products, such as PG appear to be practical approaches to the worldwide problem of subsoil acidity and infertility. PG has a gypsum content of 85-93% and has a similar ameliorating effect to gypsum on subsoil acidity and fertility (Alcordo and Rechcigl, 1993). The PG that is applied to the soil surface moves along the profile under the influence of percolating water increasing in the supply of Ca and a reduction in the Al toxicity in the subsoil (Caires et al., 1999). PG is therefore an alternative for improving the root environment in the subsoil and can be used in acidic soils as a supplement for liming (Alva et al., 1990; Mathews and Joost, 1990 and Caires et al., 2003). PG was also applied on polluted acid soils, where the potential for metal ion leaching is higher (Dijkstra et al., 2004). Leaching of accumulated metals can contaminate surface water and groundwater used for irrigation, drinking water, and wildlife habitats. These in-situ treatments provide no actual reduction in trace element concentrations. Rather, they enhance the soil’s natural attenuation mechanisms controlling metal mobility and bioavailability, thus reducing to some extent the potential toxicity of metals to humans and to the environment. PG application reduced significantly the total metal leached from polluted acidic soil (Campell et al., 2006) with longer metal travel times. It was estimated that the mass of cadmium (Cd), copper (Cu) and lead (Pb) leached from the soil would be reduced by more than 60 to 99% with the addition of PG. This approach reduces waste disposal through revalorization of industrial wastes into industrial co-products (Lombi et al., 2002). There are more than 52,000 ha in northern Tunisia characterized by acid forest soils (Ennejah et al., 2010). Therefore, the effect of Tunisian PG on soil acidity was investigated in a column leaching experiment and in an in situ field experiment under natural conditions. This work explores as a test case the possibility of enhancement of woodland acid soil of the region of Nefza (Tunisia) by PG amendments and to evaluate its effects on soil properties and on levels of Cd mobility and transfer into the soil profile.
-
Materials and methods
-
Soil sample and amendments
-
The soil used for the leaching study was collected from woodland at Nefza, where the mean annual temperature and precipitation are 5.0°C and 600 mm, respectively. Soil was taken from the top layer (0-20 cm), air-dried and ground to pass a 2-mm sieve. The soil is Mediterranean red as the local Béja soils, found also in Bou Kornine and Jebel Zaghouan and developed on Jurassic limestone or on glacis of the Quaternary. It is fine textured with particle size distribution of 35% for Ø < 2 mm (clay), 32.3% for 2-50 mm particles (silt) and 32.7 % of Ø> 50 mm (sand). The woodland was undisturbed, unfertilized and under a natural vegetation of cork oak (Quercus suber) and herbaceous plants. The soil is slightly acid with a litter layer. The level of organic matter in the litter was high equal to 67.13%. Its pH was 6.32 (± 0.04) and had 3 mg.kg-1 of cadmium.
PG is a by-product obtained from the Tunisian phosphoric acid industry located in Sfax, Tunisia. It is generated in the wet-acid production of phosphoric acid through treatment of rock phosphate with sulfuric acid. Chemical analysis data for the PG are given in Table 1. It contains 32.8 % CaO, 44.4 % SO3, 1.69 % P2O5 and a small residue of F (0.55 %). In a physical analysis of the phosphogypsum, 40 and 80% of the particles were retained in 100 and 1000 µm-mesh sieves, respectively.
Table 1. Composition* of applied phosphogypsum (Sfar felfoul et al., 2002)
|
||||||||||
Elements |
SO3 |
Al2O3 |
CaO |
F |
Fe2O3 |
Na2O |
MgO |
P2O5 |
Cd |
|
Units |
% |
mg.kg-1 |
||||||||
|
44.4 |
0.11 |
32.8 |
0.55 |
0.03 |
0.08 |
0.01 |
1.69 |
7.5 |
|
*Element concentrations are given on a dry weight basis |
-
Column leaching experiment
Leaching columns were made of rigid polyvinyl chloride (PVC) of 25.0 cm long and 10.0 cm inside diameter. A hole of 0.5 cm diameter was made on the bottom-end for drainage. The column bottom was filled with a 3 cm layer of acid-washed sand-silica, underlain by a fine-meshed nylon to hold the soil in place. Each column was then separately filled with two soil segments, the first segment with 0.7 kg of 0-10 cm layer and the second one with 0.7 kg of 10-20 cm layer of Nefza soil. Only the first segment was amended with PG in eight treatments, enriched or not by litter. The PG application rates with 1.3, 2.6 or 5.2 g PG.kg-1 surface soil are equivalent to 2, 4 and 8 t.ha-1 respectively; they are those commonly used in the field. One litter application rate (12.6 g.kg-1) is used and was estimated as the equivalent to the natural quantity under woodland. (T): control with no amendment; (D2): 1.3 g PG.kg-1 ; (D4): 2.6 g PG.kg-1; (D8): 5.2 g PG.kg-1; (TL): Litter; (D2L): Litter + 1.3 g PG kg-1 (D4L): Litter + 2.6 g PG kg-1 and (D8L): Litter + 5.2 g PG.kg-1. The irrigation volume took into account 1/12 of the annual average rainfall of Nefza area which corresponds to 1350 ml of rainwater in each column. This water volume was added in three weeks: 450 ml/column/irrigation. Four replicates per treatment were used. The soil was not disturbed during the experiment. Leachates were analyzed for Cd2+ and available P.
-
Field experiment
A field experiment was set up in the same field from where the soil had been collected for the column experiment. The experimental design was a randomized complete block. There were four treatments (added at one time application) equivalent to 2, 4 and 8 Mg PG.ha-1, on air-dry weight basis, with no PG as control, replicated three times using 1.0 m x 1.0 m plots. Treatments included two methods of tillage 1) surface PG application (S) and 2) PG incorporation and mixing with soil to a depth of 10 cm (I). In order to avoid disturbance, no crop was planted in these plots. After 6 months, soil subsamples from three depths (0-20, 20-40 and 40–60 cm) were collected from each plot. For each treatment, sub-samples were thoroughly mixed to a composite sample.
All samples were analyzed according to standard procedures of Pawels et al. (1992). The soil fraction of 2 mm was used to determine pH (1/2.5 soil water extract) using a pH meter. The soil organic matter (SOM) was determined using the Walkley–Black procedure. For Cd, soil samples were digested: 1g air-dried and powdered soil sample was taken into a Pyrex beaker and 10 ml of aqua regia was added. It was kept overnight and then heated on the hot plate for 2 hours. Then the extracts were filtered and the final volume of 100 ml was made with 2M nitric acid solution. Concentrations of Cd in the filtrates of digested soils and in the column leachates were measured by an atomic absorption spectrophotometer (AAS).
-
Statistical analyses
The effects of PG on soil pH, available P and soil total Cd at each depth were analyzed using a one-way analysis of variance (ANOVA). Significant (P=0.05) differences between means were identified using the least significant difference (LSD) test.
-
Results and discussion
-
Soil proprieties
-
Mean values of the physical characteristics of soil samples (0 – 20 cm) are shown in Table 2. Soil is a clayey silt, slightly acidic (pH-H2O = 6.5).
The soil had low CEC (< 3meq/100 g). Mean value of SOM was 4.2 %. Total soil cadmium (Cd) exceeded the permissible limit (1.4 mg.kg-1) set by the Canadian Soil Quality Guidelines (2007) which may be due to the natural source of trace element (Cd) in the soil that is the parent material alteration. In such moderate acidic pH of the soil, a great influence on the mobility and bioavailability of heavy metals occurs (Nigam et al., 2001; Jan et al., 2010). High Cd concentrations at low pH have been also reported by Kashem et al. (2006). While metals (Cu, Fe, Mn, Ni, and Zn) are very tightly bound to the soil at high pH, they are more available at low pH, and this can cause potential metal toxicities for crops in acid soils.
Table 2. Properties of soil samples collected from the study area |
|||
|
|
Depth (cm) |
|
|
0-20 |
20-40 |
40-60 |
pH |
6.48±0.29 |
6,57±0.04 |
6,63±0.15 |
SOM a (%) |
6.14±0.29 |
2.94±0.79 |
1.32±0.43 |
P (mg.kg-1) |
28.63±1.76 |
26.23±3.6 |
29.57±1.4 |
CEC (cmol(+).kg-1) |
2.59 ±0.28 |
1.33±0.1 |
Nd |
Cd (mg.kg-1) |
3.7±0.6 |
4.7±2.1 |
3.7±1.5 |
a Soil organic matter; ± Standard deviation; nd: not determined |
Statistical analysis showed that the application of PG had no effect on the amount of Cd percolated either in the presence or absence of litter. Similar results was obtained by Nisti et al. (2015) who studied in the laboratory the addition of Brazilian PG on columns filled with Brazilian typical sandy and clay acid soils with pH 3.8 and 4.9 respectively and percolated with water. Two rates of PG were applied for each soil equivalent to 4 t PG.ha-1 and 2 t PG.ha-1 for sandy and clay soil respectively and a rate of 10 times of the amount of PG necessary to achieve 50% of the soil base saturation . Results showed that PG application to the soils, even in quantities that exceeded 10 times the recommended rates, does not contribute to an enhancement of metal contents in leached solution.
|
Figure 1. Cadmium amount in leached solutions for the different treatments. T, D and DL denote control, phosphogypsum rate and mixture of litter and phosphogypsum. 2, 4 and 8 denote the PG rates of 2, 4 and 8 t PG.ha-1, respectively. |
The amount of Cd was significantly higher in the first leachates for all PG rates with or without litter. So the soil Cd in labile form seems to be rapidly dissolved and percolated (Figure 1). PG addition had no significant effects on leached Cd. But in Campbell et al. (2006) Cd transport studies in undisturbed soil columns of an acidic soil amended with PG, a significant reduction of the mass recovery of Cd in effluent from the soil columns was observed. Significant difference was obtained for the percolates indicating that the amount of leached Cd changes with the time. The travel time characteristics were affected by PGs additions with retardation values for Cd averaging 1.6 for all treated columns against 1.2 for the control columns, this difference would result in a significant delay in transport of the metals deeper into the soils or to the groundwater table.
A theoretical evaluation of the environmental consequences of a PG amendment with or without litter (Figure 2) was established for the different treatments by calculation of Cd inputs in g Cd.ha-1 soil via the different Cd sources (litter and PG), considering the concentration of Cd (mg.kg-1) in each source on dry weight basis and the Cd amount in the columns. Cd outputs were based on Cd leached amount in the study period.
|
Figure 2. Balance of cadmium for the different treatments. T, D and DL denote control, phosphogypsum rate and mixture of litter and phosphogypsum. 2, 4 and 8 denote the application rates of 2, 4 and 8 t PG.ha-1, respectively. |
For PG amended soils the amount of percolated Cd decreased as PG rates increased (Figure 2). However, the decrease is not significant. The effect of PG addition on mobility and chemical availability of Cd was not clear. For the control soils, the percolated Cd was the effect of rainfall on soil Cd. Percolation rate calculated as the ratio between Cd outputs for the three percolates and the Cd inputs for PG-amended soils varied between 12% and 30% for the highest rate equivalent to 8 t PG.ha-1 with and without litter respectively. Nisti et al. (2015) based on a column experience had reported that the Cd concentration in the leachate solutions from the PG amended soil were low (below quantification limit 13 mg.L-1). Only a part of soil Cd was affected by lixiviation since trace metals are mainly associated with solid soil components and exist in various physico-chemical forms. Thus, total concentrations of trace metals in PG amended soils and amounts of leached Cd provided little or no indication of their specific bioavailability, mobility and reactivity. Consequently, the knowledge of metal speciation and distribution between different size fractions in PG-treated soils may be useful to a better quantification of its potential environmental effects.
Six months after PG application in situ on Nefza soil, soil pH; available P and Cd contents (mg.kg-1) are compared for the 3 soil layers and for all treatments (Table 3).
Table 3. Nefza acidic soil proprieties under crock oak forest amended with phosphogypsum |
|||
|
pH |
P (mg.kg-1) |
Cd (mg.kg-1) |
Tillage (T) |
|
|
|
S |
6.34±0.37a |
27.49±5.01a |
3.94±0.82a |
I |
6.12±0.38b |
18.71±4.41b |
4.11±0.97a |
Rates (Mg/ha) (PG) |
|
|
|
0 |
6.37±0.37a |
25.10±4.47a |
4.77±0.95a |
2 |
6.20±0.50a |
25.13±5.91a |
3.83±0.91a |
4 |
6.41±0.15a |
21.40±6.08a |
3.66±0.88a |
8 |
5.94±0.28b |
20.79±4.57a |
3.83±0.74a |
Depths (cm) (D) |
|
|
|
0-20 |
6.15±0.50a |
24.29±5 .34a |
4.25±0.72a |
20-40 |
6.18±0.32a |
21.86±5.51a |
3.87±1.16a |
40-60 |
6.36±0.29a |
23.16±5.26a |
3.95±0.76a |
ANOVA |
|
|
|
T |
* |
*** |
NS |
PG |
*** |
NS |
NS |
D |
NS |
NS |
NS |
T×PG |
** |
NS |
NS |
PG×D |
NS |
NS |
NS |
T×PG×D |
NS |
** |
NS |
Means with same letter (s) are not significantly different at P<0.05, S: Surface application of PG and I: Incorporation of PG to 10 cm , * Significant at P<0.0.5, *** significant at P<0.01 NS: not significant |
Both for surface application as for incorporation of phosphogypsum, soil pH were lower in the PG-amended soils compared to the control. The incorporation of PG induced significant reduction of soil pH. Differences due to tillage were statistically significant. Furthermore, PG rates × Tillage interaction was also significant. Soil pH variation was between 0.30 and 0.50 pH units, and was no significant except for the highest rate (8 Mg.ha-1). The tendency of the highest rate of PG to decrease the soil pH, for all layers, may be due to Ca ions in CaSO4 that could displace adsorbed H which then increases the H ion activity in the soil solution. Large quantities of Ca may also displace adsorbed Al ions which could hydrolyze to produce more H ions in the soil solution, making the soil solution more acidic. These processes are known as the “salting effect”. However, because of the SO4 in CaSO4 in Tunisian PG (80%), PG has also the potential to neutralize the H ions in soil solutions. In a ligand exchange, the SO4 could replace the OH ion tied to Fe or Al oxides and hydrous oxides, mostly in the subsoil, bringing OH ions into solution. In fact, several studies (Da Costa and Crusciol, 2016; Soratto and Crusciol, 2008 and Caires et al., 2003) had reported that surface phosphogypsum application influenced the soil acidity components and observed an increase in the soil pH in the surface layers (20-40 cm) after 8 months of PG additions. The effect of PG addition still registered in 40-60 cm layer, 32 months after PG additions. A decrease in the exchangeable Al, 12 months after PG application, was also reported. In addition, aluminum precipitation could also occur with the formation of minerals. Da Costa and Crusciol (2016) reported soil acidification after long term (60 months) surface application of PG on tropical acid soils in Brazil suggesting that the acidification processes manifest through the pH values, considering the soil buffering capacity and the nutrient extraction processes. However, Li et al. (2015) reported that PG had little effect on soil pH in amended acid soils. Several reports described also a lack of crops response to the surface application of soil acidity correctives (no-till systems) in the subtropical region of Brazil (Moreira et al., 2001 and Caires et al., 2011).
The PG used in the study contained about 1.69 mg P.kg-1. The effect of the PG on soil P would be the association of its effects as P application and on the native P soil.
In the present study, the tillage method and the interaction between “Tillage × PG rates × Depths” had a significant effect on P availability (Table 3). For the control, the distribution of P is almost identical for the three layers showing an initial vertical homogeneity in available P (Figure 3). Only the treatment (8 Mg PG.ha-1) with incorporated PG increased available P in the first few centimeters (0-20 cm) in comparison with the surface application modality. Soil available P decreased globally, showing that PG tendency to increase the P retention capacity of the various soil layers (Figure 3).
(a) |
|
Figure 3. Trends in available P (mg.kg-1) by Depth, of Nefza acidic soil under crock oak forest amended with phosphogypsum (PG) with surface application (a) and with mixing of PG (b). |
This may be due to the reaction between the Ca from the PG and the soil phosphate ions which rendered the P less available. Large amounts of SO4 from the applied PG did not effectively displace phosphate in the soil exchange complex. The increased P availability with phosphogypsum described by Da Costa and Crusciol (2016) in the first 10 cm layer may be related to the competitive adsorption of sulphate and phosphate in acidic conditions (Geelhoed et al., 1997). Lee et al. (2009) reported on acid soil that the available P content in the soil increased with increasing addition of PGs associated with an increase of soil pH.
-
Effect of PG amendment on total soil cadmium under field conditions
The total concentrations (mg kg-1) of Cd in PG used in the present study were 7.5 (Table 1). The mean soil Cd concentrations ranged between 4.7 and 3.8 mg.kg-1 for the control and for treated soils respectively (Figure 4) exceeding the limit set by the Canadian Council (2007) for contaminated soils.
|
(b) |
Figure 4. Trends in soil Cd content (mg.kg-1) by Depth, of Nefza acidic soil under crock oak forest amended with PG as surface application (a) and with mixing of PG (b) |
Cd concentration for the control soil was attributed to the fact that the study area corresponds to the Triassic dome, the parent rocks are enriched with heavy metals, leading to soil contamination. Besides, the study area was near the ancient iron mine of Tamra. The local geology of the area may influence the soils. Transportation and release of minerals from their source rocks are very complicated process and involve so many variables that it makes it impossible to correctly guess the distance a particular mineral can travel. Therefore, to locate the source rocks for minerals, great efforts and investigation are needed; during which all tributaries, channels and soils must be checked for the concerned minerals, which is not an easy job. According to Dijkstra et al. (2004), the potential for metal ion leaching is greater in acid soils, while in soils near neutral pH, dissolved organic material dominates metal complexes and the leaching processes. The results showed in Figure 4 indicated that PG had no significant effect on the concentrations of Cd for the three depths and for the two tillage modalities.
Cadmium concentrations and trends were consistent with those reported by Rechcigl et al (1992) stating that an application of 20 Mg PG.kg-1 on acidic soils (pH=5.4) had no significant effect on heavy metals (Hg, Sr, Cd, Cu and Pb) concentrations of the soil. The formation of Al-hydroxyl polymers onto organic and inorganic particles and the addition of ligands such as sulfate and phosphate anions in the amendments enhanced the metal sorption capacity to the soil (Garrido et al., 2006).
-
Conclusion
The results of the column and field studies demonstrate the beneficial effects of phosphogypsum application to acid-affected soil. The applied amendment reduced the mass recovery of Cd in effluent for the column experiment. Although the difference between the treated and control column leached Cd was not large, this difference would result in a reduction of the amount of Cd travelling deeper into the soils or to the groundwater table. Therefore, the potential of PG to reduce metal leaching in polluted agricultural soils should be considered. For the field experiment PG addition had decreased soil pH and had no effect on available P except for the highest rate of 8 Mg.kg-1 with incorporated PG to 10 cm. No significant effect of PG addition on soil Cd contamination was reported even with high PG rates in these polluted soils. This study was a primary effort to estimate the possibility of using this industrial by-product as soil amendments. And further studies are needed to establish the economic feasibility based on the marginal rate of return from phosphogypsum application of such project since the fertilizer factory and PG storage are located in the southern Tunisia.
Acknowledgments
This publication is a part of the joint initiative of the National Agronomic Institute of Tunisia (INAT), Soil Science and Environment Laboratory, and the Tunisian phosphoric acid industry (GCT) (Sfax factory).
-
References
Alcordo IS, Rechcigl JE (1993) Phosphogypsum in agriculture: a review. Adv Agron Advances in Agronomy, 49, 55-118.
Alva AK, Sumner ME, Miller WP (1990) Reactions of gypsum or phosphogypsum in highly weathered acid subsoils. Soil Science Society of America Journal, 54, 993-998.
Caires EF, Blum J, Barth G, Garbuio FJ, Kusman MT (2003) Changes in chemical soil characteristics and soybean response to lime and gypsum applications in a no-tillage system. Revista Brasileira de Ciência do Solo, 27, 275-28. http://dx.doi.org/10.1590/S0100-06832003000200008.
Caires EF, Fonseca AF, Feldhaus IC, Blum J, (2001) Root growth and nutrient uptake by soybean as affected by lime and gypsum, under a no-tillage system. Revista Brasileira de Ciência do Solo, 25, 1029-1040.
Caires EF, Fonseca AF, Mendes J, Chueiri WA, Madruga EF (1999) Corn, wheat and soybean yields as a function of the changes in soil chemical characteristics due to surface application of lime and gypsum under a no-tillage system. Revista Brasileira de Ciência do Solo, 23, 315-327.
Caires EF, Joris HAW, Churka S (2011) Long-term effects of lime and gypsum additions on no-till corn and soybean yield and soil chemical properties in southern Brazil. Soil Use Management, 27, 45-553.
Campbell CG, Garrido F, Illera V, Garcia-Gonzalez MT (2006) Transport of Cd, Cu and Pb in an acid soil amended with phosphogypsum, sugar foam and phosphoric rock. Applied Geochemistry, 21, 1030-1043.
Canadian Council of Ministers of the Environment, (2007) Canadian soil quality guidelines for the protection of environmental and human health: Summary Tables. Update 7.0. On line at: http://www.ec.gc.ca/ceqg-rcqe
Lee CH, Ha BY, Lee YB, Kim PJ (2009) Effect of Alkalized Phosphogypsum on Soil Chemical and Biological Properties. Communications in Soil Science and Plant Analysis, 40, 2072-2086. DOI: 10.1080/00103620902960591
Conyers MK, Heenan DP, Mc Ghie WJ, Poile GP (2003) Amelioration of acidity with time by limestone under contrasting tillage. Soil and Tillage Research, 72 (1), 85-94.
Da Costa CH, Da CostaH M, Crusciol MCA, Crusciol, C (2016) Long-term effects of lime and phosphogypsum application on tropical no-till soybean–oat–sorghum rotation and soil chemical properties. European Jouranl of Agronomy, 74, 119-132.
Dijkstra J, Meeussen CL, Rob NJ, Comans RNJ (2004) Leaching of Heavy Metals from Contaminated Soils: An Experimental and Modeling Study. Environmental. Science and Technology, 38 (16), 4390-4395.
Enamorado Báez SM, Abril JM, Mas JL, Peria˜nez R, Polo OP, Delgado A, Quintero JM (2009) Transfer of Cd, Pb, Ra and U from phosphogypsum amended soils to tomato plants. Water Air Soil Pollution, 203, 65-77.
Garrido F, Illera V, Campbell CG, Garcia-Gonzalez MT, Gonzalez A (2006) Regulating the mobility of Cd, Cu and Pb in an acid soil with amendments of phosphogypsum, sugar foam, and phosphoric rock. European Journal of Soil Science, 57, 95-105.
Geelhoed JS, Hiemstra T, Van Riemsdijk WH (1997) Phosphate and sulfate adsorption on goethite: single anion and competitive adsorption. Geochimica. Cosmo chimica. Acta, 61, 2389-2396.
Jan FA, Ishaq M, Khan S, Ihsanullah I, Ahmad I, Shakirullah M (2010) A comparative study of human health risks via consumption of food crops grown on wastewater irrigated soil (Peshawar) and relatively clean water irrigated soil (Lower Dir). Journal of. Hazardous. Materials, 179, 612-621.
Li JY, Liu ZD, Zhao WZ, Masud MM, Xu RK (2015) Alkaline slag is more effective than phosphogypsum in the amelioration of subsoil acidity in an Ultisol profile. Soil and & Tillage Research, 149, 21-32.
Kashem MDA, Singh BR, Kawai S (2006) Mobility and distribution of cadmium, nickel and zinc in contaminated soil profiles from Bangladesh. Nutrient. Cycling in. Agroecosystems, 77, 187-198.
Liu J, Hue NV (2001) Amending subsoil acidity by surface applications of gypsum, lime, and composts. Communications in Soil Science and Plant Analysis, 32 (13&14), 2117-2132.
Lombi E, Zhao FJ, Zhang G, Sun B, Filtz W, Zhang H, McGrath SP (2002) In situ fixation of metals in soils using bauxite residue: chemical assessment. Environmental pollution, 118, 435-443.
Nisti MB, Saueia CR, Malheiro LH, Groppo GH, Mazzilli BP (2015) Lixiviation of natural radionuclides and heavy metals in tropical soils amended with phosphogypsum. Journal of Environmental Radioactivity, 144, (44), 120-126.
Mathews BW, Joost RE (1990) The effects of leaching surface‐applied amendments on subsoil aluminum and alfalfa growth in a Louisiana Ultisol. Communications in Soil Science and Plant Analysis, 21, (7&8), 567-581.
Moreira SG, Kiehl JC, Prochnow LI, Pauletti V (2001) Liming under no-tillage and effects on soil acidity, soil nutrient availability and corn and soybean yield. Revista Brasileira de Ciência do Solo, Rev. Bras. Ciênc. Solo 25, 71-81.
Nigam R, Srivastava S, Prakash S, Srivastav MM (2001) Cadmium mobilization and plant availability-the impact of organic acids commonly exuded from roots. Plant and Soil, 230, 107-113.
Pauwels JM, Van Ranst E, Verloo M, Mvondo ZA (1992) Manuel de laboratoire de pédologie: Méthodes d’analyse de sol et des plantes. Equipement, gestion des stocks de verreries et produits chimiques. Publication agricole. AGCD, Bruxelles. 250 p.
Rechcigl JE, Alcordo S, Roessler CE, Littell RC (1992) Influence of phosphogypsum on forage yield and quality, and on the environment in a typical Florida spodosol soil. Progress Report, Project 89-01-085R. Florida Institute of Phosphate Research, Bartow, FL.
Sfar Felfoul H, Clastres P, Carles-Gibergues A, Ben Ouezdou M (2002) Propriétés et perspectives d'utilisation du phosphogypse ; l'exemple de la Tunisie. In: Proceedings of International Symposium on Environmental Pollution Control and Waste Management.7 - 10 Janvier 2002, Tunis. Tunisie. pp: 510-520.
Singh AL, Joshi YC, Chaudhari V, ZalaZella PV (1990) Effect of different sources of iron and sulfur on leaf chlorosis, nutrient yield of groundnut. FertFertilizer. Research Journal, .24, 85-96.
Soratto RP, Crusciol CAC (2008) Dolomite and phosphogypsum surface application effects on annual crops nutrition and yield. Agron. J.Agronomy Journal, 100, 261-270. , http://dx.doi.org/10.2134/agronj2007.0120.
Sumner ME, Shahandeh H, Bouton, J, Hammel J (1986) Amelioration of an Acid Soil Profile through Deep Liming and Surface Application of Gypsum. Soil Science Society of America Journal, 50 (5), 1254-1258.
Sumner ME, Noble AD (2003) Soil acidification: the world story. In: Rengel, Z. (Ed.), Handbook of Soil Acidity. Marcel Dekker, New York, pp. 1-28.