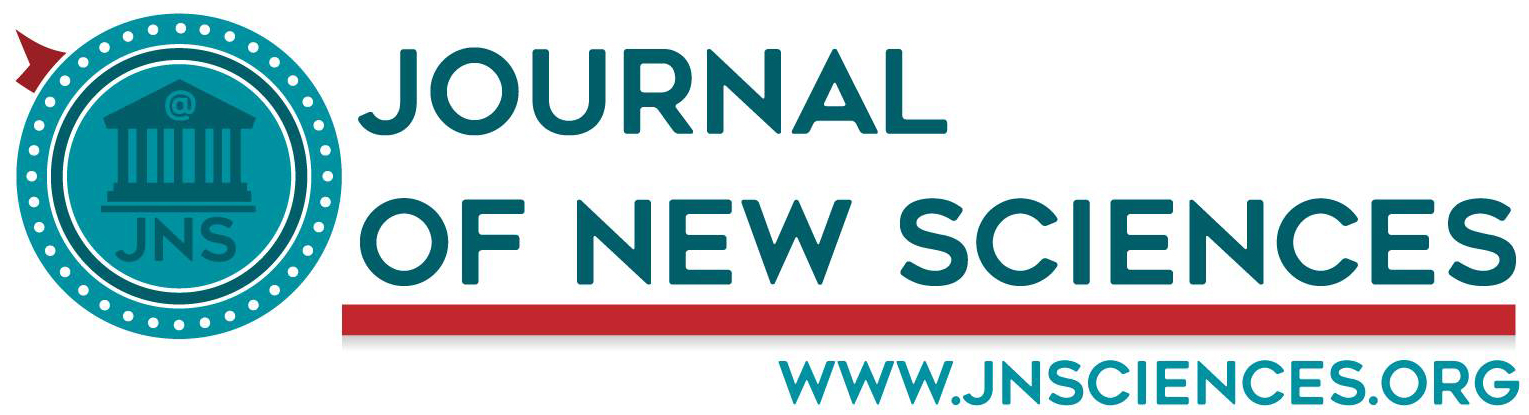
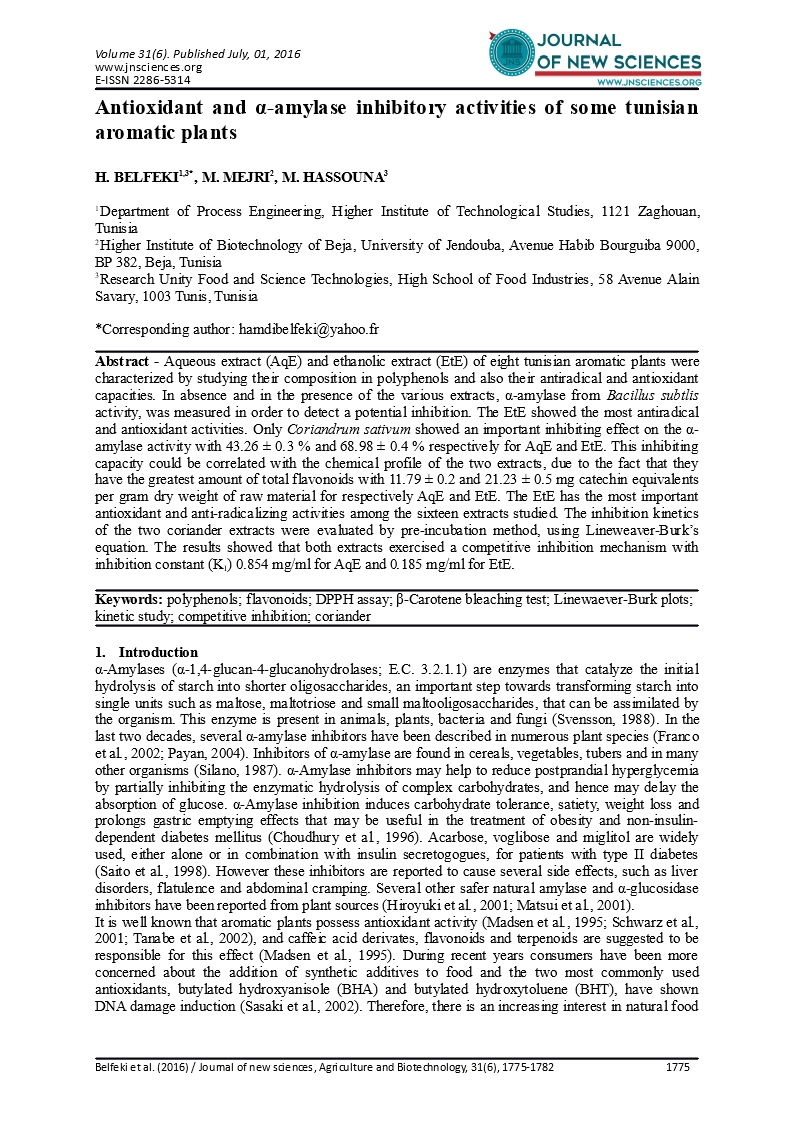
- Category: Volume 31
- Hits: 6565
Antioxidant and α-amylase inhibitory activities of some tunisian aromatic plants
H. Belfeki1,3*
M. Mejri2
M. Hassouna3
1Department of Process Engineering, Higher Institute of Technological Studies, 1121 Zaghouan, Tunisia
2Higher Institute of Biotechnology of Beja, University of Jendouba, Avenue Habib Bourguiba 9000, BP 382, Beja, Tunisia
3Research Unity Food and Science Technologies, High School of Food Industries, 58 Avenue Alain Savary, 1003 Tunis, Tunisia
Abstract - Aqueous extract (AqE) and ethanolic extract (EtE) of eight tunisian aromatic plants were characterized by studying their composition in polyphenols and also their antiradical and antioxidant capacities. In absence and in the presence of the various extracts, α-amylase from Bacillus subtlis activity, was measured in order to detect a potential inhibition. The EtE showed the most antiradical and antioxidant activities. Only Coriandrum sativum showed an important inhibiting effect on the α-amylase activity with 43.26 ± 0.3 % and 68.98 ± 0.4 % respectively for AqE and EtE. This inhibiting capacity could be correlated with the chemical profile of the two extracts, due to the fact that they have the greatest amount of total flavonoids with 11.79 ± 0.2 and 21.23 ± 0.5 mg catechin equivalents per gram dry weight of raw material for respectively AqE and EtE. The EtE has the most important antioxidant and anti-radicalizing activities among the sixteen extracts studied. The inhibition kinetics of the two coriander extracts were evaluated by pre-incubation method, using Lineweaver-Burk’s equation. The results showed that both extracts exercised a competitive inhibition mechanism with inhibition constant (KI) 0.854 mg/ml for AqE and 0.185 mg/ml for EtE.
Keywords: polyphenols; flavonoids; DPPH assay; β-Carotene bleaching test; Linewaever-Burk plots; kinetic study; competitive inhibition; coriander
-
Introduction
α-Amylases (α-1,4-glucan-4-glucanohydrolases; E.C. 3.2.1.1) are enzymes that catalyze the initial hydrolysis of starch into shorter oligosaccharides, an important step towards transforming starch into single units such as maltose, maltotriose and small maltooligosaccharides, that can be assimilated by the organism. This enzyme is present in animals, plants, bacteria and fungi (Svensson, 1988). In the last two decades, several α-amylase inhibitors have been described in numerous plant species (Franco et al., 2002; Payan, 2004). Inhibitors of α-amylase are found in cereals, vegetables, tubers and in many other organisms (Silano, 1987). α-Amylase inhibitors may help to reduce postprandial hyperglycemia by partially inhibiting the enzymatic hydrolysis of complex carbohydrates, and hence may delay the absorption of glucose. α-Amylase inhibition induces carbohydrate tolerance, satiety, weight loss and prolongs gastric emptying effects that may be useful in the treatment of obesity and non-insulin-dependent diabetes mellitus (Choudhury et al., 1996). Acarbose, voglibose and miglitol are widely used, either alone or in combination with insulin secretogogues, for patients with type II diabetes (Saito et al., 1998). However these inhibitors are reported to cause several side effects, such as liver disorders, flatulence and abdominal cramping. Several other safer natural amylase and α-glucosidase inhibitors have been reported from plant sources (Hiroyuki et al., 2001; Matsui et al., 2001).
It is well known that aromatic plants possess antioxidant activity (Madsen et al., 1995; Schwarz et al., 2001; Tanabe et al., 2002), and caffeic acid derivates, flavonoids and terpenoids are suggested to be responsible for this effect (Madsen et al., 1995). During recent years consumers have been more concerned about the addition of synthetic additives to food and the two most commonly used antioxidants, butylated hydroxyanisole (BHA) and butylated hydroxytoluene (BHT), have shown DNA damage induction (Sasaki et al., 2002). Therefore, there is an increasing interest in natural food additives, such as aromatic plants or aromatic plants extracts, which can function as natural antioxidants besides seasoning the food. In addition, polyphenols are known to inhibit the activity of digestive enzymes such as amylase, glucosidase, pepsin, trypsin and lipase, and the subject has been studied extensively (Rohn et al., 2002). In this study, eight tunisian aromatic plants were selected on the basis of their use in traditional medicines and kitchen through north africa. Extracts of these species were tested for their antioxidant and anti α-amylase activity.
-
Materials and methods
-
Preparation of extracts
-
Each part used of Trigonella foenum graecum, Coriandrum sativum, Helienthus annuus, Allium sativum, Artemisia herba alba, Capparis spinosa, Ocimum basilicum and Jenupersus phoeniceae was cut or sliced into small pieces and air-dried in the shade. The dried samples were then ground to a fine powder using a grinder. Maceration of crude powder was carried out in a one-step extraction (batch mode). Dried sample (10 g) was extracted with 100 ml of distilled water or ethanol. The mixture was agitated during 30 min and kept 24 h in -4 °C in darkness. Finally the mixture was filtered through a No. 1 Whatman paper.
-
Determination of total phenolics and total flavonoids contents
The total phenolics content (TPC) was determined with the Folin-Ciocalteu reagent at 760 nm (Lister& Wilson, 2001). The results were expressed as mg gallic acid equivalents per gram dry weight of raw material (mg GAE/ g dry weight). The total flavonoids content (TFC) was determined by spectrophotometric assay at 510 nm (Dewanto et al., 2002). The results were expressed as mg catechin equivalents per gram dry weight of raw material (mg CE/ g dry weight).
-
DPPH radical scavenging assay
The capacity of prepared extracts to scavenge the 'stable' free radical 2,2-diphenyl-1-picrylhydrazyl (DPPH) was monitored (Hatano et al., 1988). The radical scavenging activity (RSA) was calculated as a percentage of DPPH discoloration using the equation:
|
(1) |
where AE is the absorbance of the solution when an extract has been added, and AD is the absorbance of the DPPH solution with nothing added. IC50 values, which correspond to the concentration of extracts that caused a 50% neutralization of DPPH, were calculated from the plot of percent DPPH scavenging versus concentration.
-
β-Carotene bleaching test
The antioxidant activity of extracts was evaluated according to a version of the β-carotene bleaching method (Pratt, 1980). The antioxidant activity (AA) was evaluated using the following formula:
|
(2) |
where and
are the absorbance values measured at zero time of the incubation for test sample and control, respectively.
and
are the absorbance measured in the test sample and control, respectively at (t) time of incubation.
-
α-Amylase inhibition screening
Starch in a 0.1 M Tris-HCl buffer (pH 6) was used as a substrate solution (Bernfeld, 1955). In this experiment (non-pre-incubation method), plant extract and starch were mixed. The reaction was started by the addition of enzyme. The tubes were incubated at 60 °C for 10 min. Final concentrations in the incubation mixture were plant extract, 0.4 mg/ml, 0.8 mg/ml starch and 0.02 mg/ml enzyme. The reaction was terminated by adding 1 ml of dinitrosalicylic acid solution (DNS) and boiled for 10 min in a boiling water bath, and 1 ml of distilled water was added. Enzyme activity (EA) was quantified by measuring the reducing sugar released as the glucose standard from the substrate per min and per mg of enzyme at 540 nm (1 EA = 1 µmol eq glucose/min.mg). The α-amylase inhibition (αAI) was calculated according the following formula:
|
(3) |
Where A is the activity without inhibitor, a : the negative control without inhibitor, B : the activity with inhibitor, and b is the negative control with inhibitor.
-
Kinetics of enzyme inhibition
In the second experiment (a pre-incubation method), α-amylase was pre-incubated 10 min at room temperature with the plant extract and the reaction was started by the addition of starch solution at different concentrations (0.2 - 2 mg/ml). The final concentration of the α-amylase inhibitor was 0.4 mg/ml. The rest of the procedure was carried out as in the first experiment. The Michaelis constant (KM), inhibition constant (KI), maximal velocity (Vm) and the inhibition mode was determined using Lineweaver–Burk equation:
|
(4) |
-
Statistical analysis
All determinations were made in triplicate and data is reported as mean ± SD. Data were analyzed using ANOVA variance analysis, Minitab 2012 Version 16.2.3 statistical software (Minitab Inc., Pennysalvania, USA). A probability value at P ≤ 0.05 was considered statistically significant.
-
Results and discussion
-
Amount of total phenolics and flavonoids
-
The amount of total phenolics varied in the different extracts as shown in table 1. The highest total phenolic levels were detected when ethanol was used for extraction. Ethanol extracts with highest polyphenol content were Trigonella foenum graecum with 68.41 mg GAE/ g dry weight, followed by Coriandrum sativum with 49.09 mg GAE/ g dry weight. The highest total flavonoids levels were detected when ethanol was used for extraction, except for Ocimum basilicum. The plants with highest flavonoids content by ethanol extraction were Coriandrum sativum with 21.23 mg CE/ g dry weight, followed by Artemisia herba alba with 11.63 mg CE/ g dry weight. When extracts were obtained by distilled water, Coriandrum sativum also had the highest flavonoids content with 11.79 mg CE/ g dry weight, followed by Ocimum basilicum with 11.65 mg CE/ g dry weight. In both cases, Coriandrum sativum was the richest in flavonoids. The amount of total phenolics compounds, in all tested extracts, was higher than those of some Asian vegetables (Kaur& Kapoor, 2002), Colombian Amazonian plants (Lizcano et al., 2010), some cereals such as finger millet (Chethan & Malleshi, 2007) and barley (Anwar et al., 2010) but lower than those of some plant species from the Canadian prairies (Amarowicz et al., 2004).
-
DPPH radical scavenging assay
Table 1. Total phenolics and flavonoids contents |
||||||
Species |
Part used |
TPC |
TFC |
|||
Aq E |
Et E |
Aq E |
Et E |
|||
Trigonella foenum graecum |
Seeds |
56.76 ± 1.5 |
68.41 ± 1.3 |
4.21 ± 0.2a |
6.14 ± 0.1 |
|
Coriandrum sativum |
Seeds |
27.38 ± 1.2b |
49.09 ± 0.8A |
11.79 ± 0.2b |
21.23 ± 0.5 |
|
Helienthus annuus |
Seeds |
37.78 ± 0,8a |
45.25 ± 0.9AB |
3.06 ± 0.1 |
4.51 ± 0.1 |
|
Allium sativum |
Bulbs |
40.85 ± 0.5a1 |
40.12 ± 0.61 |
11.25 ± 0.4bc1 |
10.55 ± 0.3A1 |
|
Artemisia herba alba |
Aerial part |
30.1 ± 0.6b |
34.75 ± 0,4 |
10.48 ± 0.1c |
11.63 ± 0.3A |
|
Capparis spinosa |
Aerial part |
38.78 ± 1.1a |
44.6 ± 0.7B |
3.99 ± 0.1a |
10.1 ± 0.4A |
|
Ocimum basilicum |
Aerial part |
18.5 ± 1,1c |
24.38 ± 0.5C |
11.65 ± 0.3b |
3.14 ± 0.1 |
|
Jenupersus phoeniceae |
Aerial part |
17.22 ± 0,9c |
23.66 ± 1.0C |
10.04 ± 0.2c |
11.2 ± 0.3A |
|
TPC : values are expressed as mg GAE/ g dry weight (means ± standard deviation of three measurements). TFC : values are expressed as mg CE/ g dry weight (means ± standard deviation of three measurements). For each solvent, values in the same column with different letters are significantly different (P ≤ 0.05). For TPC or TFC, values in the same line with same subscript numbers are not significantly different (P ≤ 0.05). |
The IC50 values of the crude ethanolic and aqueous extracts from the eight plant species were examined as shown in figure 1.
|
Figure 1. Radical scavenging activity of aqueous and ethanolic extracts. For each solvent, values with same letters are not significantly different (P ≤ 0.05) |
The extracts of all the tested aromatic plant materials possessed free radical scavenging properties, but to varying degrees. Using ethanol as solvent for extraction technique, samples showed better DPPH scavenging activity. A maximum scavenging activity was offered by ethanolic extract of Trigonella foenum graecum, followed by ethanolic extract of Coriandrum sativum. DPPH scavenging activity, in all tested extracts, was higher than those of horsetail Equisetum spp. (Amarowicz et al., 2004), Glucagel β-glucan of barley extracted with acetone, methanol and ethanol (Thondre et al., 2011), 0.1 mM ascorbic acid (Nagai et al. 2003), but lower than those of some medicinal plant from Pakistan (Sultana et al., 2009).
-
β-Carotene bleaching test
The antioxidant activity of ethanolic and aqueous extracts was also evaluated, in comparison with synthetic antioxidant BHT, by β-carotene/linoleic acid bleaching method as shown in figure 2. Extraction in ethanol was found to be more efficient than water in extracting the antioxidants present in the tunisian aromatic plants.
a |
b |
Figure 2. Antioxidant activity of aqueous (a) and ethanolic (b) extracts.
|
A large variation in the antioxidant activities, ranging from as high as 78 % in Coriandrum sativum ethanolic extract to as low as 10% in Ocimum basilicum aquous extract, was observed. Except Ocimum basilicum, AA in all tested extracts is higher than some Asian vegetables like Allium cepa, Pisum sativum, Capsicum anunum, Phaseolus vulgarlis, Brassica rapa, Dolichus lablab, Cucumis sativus, Praecitrullus vulgaris var. fistulosius, Brassica oleracea var. botrytis, Raphanus sativus and Cucumis utilissimus (Kaur& Kapoor, 2002).
-
α-Amylase inhibition screening
To detect a potential antidiabetic activity, α-amylase inhibition by 16 tunisian aromatic plants extracts was quantified by non-preincubation method as shown in table 2. Only Coriandrum sativum L. presented an important inhibitory effect in aqueous extract and in ethanolic extract. This inhibitory potency could be correlated with chemical profile of both extracts of Coriandrum sativum. Firstly, the two extracts are richer in total flavonoids. On the other hand, ethanolic extract has a greater antioxidant activity and second best free radical scavenging activity. These properties could explain the hypoglycemic activity of coriander demonstrated in many pharmacological studies (Gray & Flatt, 1999; Chithra et al., 1999).
Table 2. α-Amylase inhibition of 16 tested extracts. |
||
Species |
α-amylase inhibition (%) |
|
Aqueous extract |
Ethanolic extract |
|
Trigonella foenum graecum |
9.93 ± 0.5de |
2.99 ± 0.1 |
Coriandrum sativum |
43.26 ± 0.3 |
68.98 ± 0.4 |
Helienthus annuus |
8.57 ± 0.3cd |
7.07 ± 0.1A |
Allium sativum |
-0.13 ± 0.1 |
15,23 ± 0,4 |
Artemisia herba alba |
5.71 ± 0.1a |
11.97 ± 0.2 |
Capparis spinosa |
7.61 ± 0.2bc |
3.81 ± 0.1 |
Ocimum basilicum |
6.80 ± 0.3ab1 |
7.21 ± 0.2A1 |
Junipersus phoeniceae |
11.70 ± 0.4e |
9.25 ± 0.3 |
For each solvent, values in the same column with different letters are significantly different (P ≤ 0.05). Values in the same line with same subscript numbers are not significantly different (P ≤ 0.05). |
The phenolic compounds may play a key role in the inhibition of starch digesting enzymes. Depending on the structure, the phenolics react with proteins/enzymes and alter various properties of biopolymers such as the molecular weight, solubility and in vitro digestibility. It has also been shown that the decrease in enzyme activity depends on the concentration as well as the number and position of hydroxyl groups of the phenolics (Rohn et al., 2002).
-
Kinetics of enzyme inhibition
Kinetic studies were performed using the Michaelis–Menten and LB derivations to identify the mode of inhibition of coriander extracts. In the presence of crude aqueous and ethanolic extracts, straight lines were intercepted at a single point on the vertical axis indicating competitive inhibition as shown in figure 3.
|
Figure 3. α-Amylase Lineweaver-Burk plots in presence of coriander extracts. |
Coriandrum sativum extracts affect α-amylase by competing with the substrate to bind to the active site of the enzyme. In this case, inhibitor can only bind to free enzyme decreasing affinity enzyme for its substrate. In fact, in the presence of aqueous and ethanolic extracts, maximum velocity (Vmax) remained constant but constant Michaeils (KM) increased as shown in table 3. An inhibitory constant (KI) value were obtained with following formula:
|
(5) |
Ethanolic extract showed most important inhibitory effect than aqueous extract. This can be explained by its higher polyphenol and flavonoids content but also by its greater antioxidant and radical scavenging activity. This bioactivity results from the presence of large number of compounds identified in coriander, including flavonoids (quercetin and isoquercetin), polyphenols (rutin, caffeic acid derivatives, ferrulic acid, gallic acid and chlorogenic acid) and β-carotenoids (Melo et al., 2003). Synergy between phenolics may play a role in mediating amylase inhibition, and therefore have the potential to contribute to the management of type 2 Diabetes mellitus, which is characterized by high blood glucose levels (Saito et al., 1998; Toeller, 1994). Polyphenols may act as inhibitors of amylase and glucosidase (similar to acarbose, miglitol and voglibose) leading to a decrease in post-prandial hyperglycemia (Bailey, 2001).
Table 3. α-Amylase kinetic parameters in presence of coriander extracts. |
|||
Parameter |
Control |
Aqueous extract |
Ethanolic extract |
Vm (µmol EG/min.mg) |
19.23 |
19.01 |
19.08 |
KM (mg/ml) |
0.94 |
1.38 |
2.97 |
KI (mg/ml) |
- |
0.854 |
0.185 |
Inhibition mode |
- |
Competitive |
Competitive |
-
Conclusion
In this study, it was shown that solvent polarity significantly affects the amount of total polyphenols and flavonoids, antioxidant capacity, radical scavenging activity and inhibitory potential of α-amylase. Tunisian aromatic plants tested have varied phytochemical profiles. The extracts obtained from Trigonella foenum graecum and Coriandrum sativum were rich in polyphenols and flavonoids. Pharmacological studies have demonstrated this antioxidant activity of Coriandrum sativum (Melo et al., 2003; Ramadan et al., 2003; Bajpai et al., 2005) and Trigonella foenum graecum (Kaviarasan et al., 2007), so they can be used as preservative ingredients in food industry if any resulting organoleptic effects are acceptable. Aqueous and ethanolic extract from coriander showed an important inhibitory effect on the α-amyalse by competitive mechanism.
Thus, we have described edible plant-based amylase inhibitors for modulation of carbohydrate breakdown and regulation of glycemic index of foods. Our results provide a scientific rationale for the use of coriander in pharmaceutical industry as a low cost nutrient useful in reducing the chronic pathologies such as diabetes mellitus. The inhibition of starch digestive enzymes by dietary phenolics may represent a biochemical rationale or mechanism for delivering some of the health benefits attributed to a diet rich in aromatic plants.
Acknowledgments
We are very grateful to Dr. Issam Smaïli (National Institute of Applied Science and Technology, Tunis, Tunisia) for providing us with enzyme and to Mrs. Fatma Souissi (Center of Biotechnology, Borj Cedria, Tunisia) for his help. This study received financial support from Ministry of Higher Education, Scientific Research and Information and Communication Technologies, Tunisia.
-
References
Amarowicz, R., Pegg, R.B., Rahimi-Moghaddam, P., Barl, B., & Weil, J.A. (2004): Free-radical scavenging capacity and antioxidant activity of selected plant species from the Canadian prairies, Food Chemistry, 84, 551–562.
Anwar, F., Abdul Qayyum, H.F., Hussain, A.I., & Iqbal, S. (2010): Antioxidant activity of 100% and 80% methanol extracts from barley seeds (Hordeum vulgare L.): stabilization of sunflower oil, Grasas y aceites, 61, 237–243.
Bailey, C.J. (2001): New approaches to the pharmacotherapy of diabetes, Pickup, J.C. & William, G. (Eds), Text book of diabetes, Blackwell Science Ltd, UK, 73.1–73.2..
Bajpai, M., Mishra, A., & Prakash, D. (2005): Antioxidant and free radical scavenging activities of some leafy vegetables, International Journal of Food Sciences & Nutrition, 56, 473–481.
Bernfeld, P. (1955): Amylases, alpha and beta, Colowick, S.P., Kaplan, N.O. (Eds), Methods in Enzymology, Academic Press, New York, 149–158.
Chethan, S., & Malleshi, N.G. (2007): Isolation and characterization of finger millet polyphenols, Food Chemistry, 105, 862-870.
Chithra, V., & Leelamma, S. (1999): Coriandrum sativum-mechanism of hypoglycemic action, Food Chemistry, 67, 229–231.
Choudhury, A., Maeda, K., Murayama, R. & Dimagno, E.P. (1996): Character of a wheat amylase inhibitor preparation and effects on fasting human pancreaticobiliary secretions and hormones, Gastroenterology, 111, 1313–1320.
Dewanto, V., Wu, X., Adom, K.K., & Liu, R.H. (2002): Thermal processing enhances the nutritional value of tomatoes by increasing total antioxidant activity, J. Agric. Food Chem., 50, 3010–3014.
Franco, O.L., Rigden, D.J., Melo, F.R., & Grossi-de-Sa, M.F. (2002): Plant a-amylase inhibitors and their interaction with insect a-amylases, Eur. J. Biochem., 269, 397–412.
Gray, A.M., & Flatt, P.R. (1999): Insulin-releasing and insulin-like activity of the traditional anti-diabetic plant Coriandrum sativum (coriander), British Journal of Nutrition, 81, 203–209.
Hatano, T., Kagawa, H., Yasuhara, T., & Okuda, T. (1988): Two new flavonoids and other constituents in cicore root : their relative astringency and radical scavenging effects, Chem. Pharm. Bull., 36, 2090–2097.
Hiroyuki, F., Tomohide, Y. & Kazunori, O. (2001): Efficacy and safety of Touchi extract, an alpha-glucosidase inhibitor derived from fermented soya beans, in non-insulin dependent diabetes mellitus, Journal of Nutritional Biochemistry, 12, 351–356.
Kaur, C., & Kapoor, H.C. (2002): Anti-oxidant activity and total phenolic content of some Asian vegetables, International Journal of Food Science and Technology, 37, 153–161.
Kaviarasan, S., Naik, G.H., Gangabhagirathi, R., Anuradha, C.V., & Priyadarsini, K.I. (2007): In vitro studies on antiradical and antioxidant activities of fenugreek (Trigonella foenum graecum) seeds, Food Chem., 103, 31–37.
Lister, E. & Wilson, P. (2001): Measurement of total phenolics and ABTS assay for antioxidant activity (personal communication), Lincoln, New Zealand: Crop Research Institute.
Lizcano, J.L., Bakkali, F., Ruiz-Larrea, M.B., & Ruiz-Sanz, J.I. (2010): Antioxidant activity and polyphenol content of aqueous extracts from Colombian Amazonian plants with medicinal use, Food Chemistry, 119, 1566–1570.
Madsen, H.L. & Bertelsen G. (1995): Spices as antioxidants, Trends in Food Science & Technology, 6, 271–277.
Matsui, T., Ueda, T., Oki, T., Sugita, K., Terhara, N., & Matsumoto, K. (2001): Alphaglucosidase inhibitory action of natural acylated anthocyanins. I. Survey of natural pigments with potent inhibitory activity, Journal of Agricultural Food Chemistry, 49, 1948–1951.
Melo, E.A., Bion, F.M., Filho, J.M., & Guerra, N.B. (2003): In vivo antioxidant effect of aqueous and etheric coriander (Coriandrum sativum L.) extracts, European Journal of Lipid Science and Technology, 105, 483–487.
Nagai, T., Inoue, R., Inoue, H., & Suzuki, N. (2003): Preparation and antioxidant properties of water extract of propolis, Food Chemistry, 80, 29–33.
Payan, F. (2004): Structural basis for the inhibition of mammalian and insect alpha amylases by plant protein inhibitors, Biochim. Biophys. Acta, 1696, 171–180.
Pratt, D.E. (1980): Natural antioxidants of soybean and other oil-seeds, Simic, M.G., & Karel, M. (Eds), Autoxidation in food and biological systems, Plenum Press, New York, 283–292.
Ramadan, M.F., Kroh, L.W., & Morsel, J.T. (2003): Radical scavenging activity of black cumin (Nigella sativa L.), coriander (Coriandrum sativum L.), and niger (Guizotia abyssinica Cass.) crude seed oils and oil fractions, Journal of Agricultural & Food Chemistry, 51, 6961–6969.
Rohn, S., Rawel, H.M., & Kroll, J. (2002): Inhibitory effects of plant phenols on the activity of selected enzymes, Journal of Agricultural Food Chemistry, 50, 3566–3571.
Saito, N., Sakai, H., Sekihara, H. & Yajima, Y. (1998): Effect of an α-glucosidase inhibitor (voglibose), in combination with sulphonyl urea, on glycemic control in type 2 diabetes patients, Journal of International Medical Research, 26, 219–232.
Saito, N., Sakai, H., Sekihara, H., & Yajima, Y. (1998): Effect of an α-glucosidase inhibitor (voglibose), in combination with sulphonilureas, on glycemic control in type 2 diabetes patients, Journal of International Medical Research, 26, 219–232.
Sasaki, Y.F., Kawaguchi, S., Kamaya, A., Ohshita, M., Kabasawa, K., Iwama, K., Taniguchi, K., & Tsuda, S. (2002): The comet assay with 8 mouse organs: Results with 39 currently used food additives, Mutation Research/Genetic Toxicology and Environmental Mutagenesis, 519, 103–119.
Schwarz, K., Bertelsen, G., Nissen, L.R., Gardner, P.T., Heinonen, M.I., Hopia, A., Huynh-Ba, T., Lambelet, P., McPhail, D., Skibsted, L.H. & Tijburg, L. (2001): Investigation of plant extracts for the protection of processed foods against lipid oxidation. Comparison of antioxidant assays based on radical scavenging, lipid oxidation and analysis of the principal antioxidant compounds, European Food Research and Technology, 212,319–328.
Silano, V. (1987): Alpha-amylase inhibitors, Kruger, J., Lineback, D. (Eds), Enzymes and Their Role in Cereal Technology, American Association of Cereal Chemists, St Paul, MN., 141–199.
Sultana, B., Anwar, F., & Ashraf, M. (2009): Effect of extraction solvent/technique on the antioxidant activity of selected medicinal plant extracts, Molecules, 14, 2167–2180.
Svensson, B. (1988): Regional distant sequence homology between amylases, K-glucosidases and transglucanosylases, FEBS Lett., 230, 72–76.
Tanabe, H., Yoshida, M. & Tomita, N. (2002): Comparison of the antioxidant activities of 22 commonly used herbs and spices on the lipid oxidation of pork meat, Animal Science Journal, 73, 389–393.
Thondre, P.S., Ryan, L., & Henry, C.J.K. (2011): Barley β-glucan extracts as rich sources of polyphenols and antioxidants, Food Chemistry, 126, 72–77.
Toeller, M. (1994): α-Glucosidase inhibitors in diabetes: Efficacy in NIDDM subjects, European Journal of Clinical Investigation, 24, 31–35.